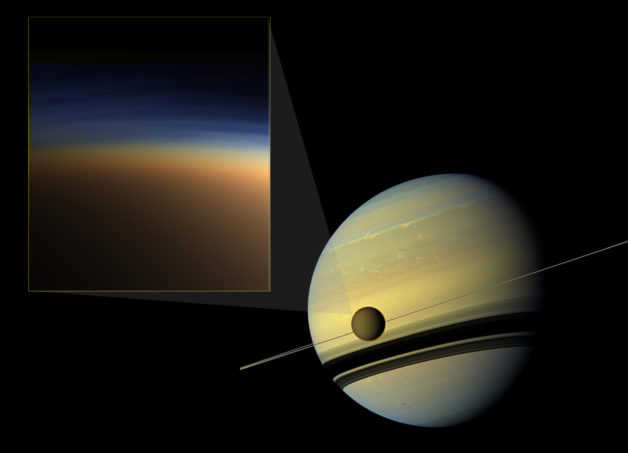
The atmospheric haze of Titan, Saturn’s largest moon (pictured here along Saturn’s midsection), is captured in this natural-color image (box at left). A study that involved experiments at Berkeley Lab’s Advanced Light Source has provided new clues about the chemical steps that may have produced this haze. (Credits: NASA Jet Propulsion Laboratory, Space Science Institute, Caltech)
Saturn’s largest moon, Titan, is unique among all moons in our solar system for its dense and nitrogen-rich atmosphere that also contains hydrocarbons and other compounds, and the story behind the formation of this rich chemical mix has been the source of some scientific debate.
Now, a research collaboration involving scientists in the Chemical Sciences Division at the Department of Energy’s Lawrence Berkeley National Laboratory (Berkeley Lab) has zeroed in on a low-temperature chemical mechanism that may have driven the formation of multiple-ringed molecules – the precursors to more complex chemistry now found in the moon’s brown-orange haze layer.
The study, co-led by Ralf Kaiser at the University of Hawaii at Manoa and published in the Oct. 8 edition of the journal Nature Astronomy, runs counter to theories that high-temperature reaction mechanisms are required to produce the chemical makeup that satellite missions have observed in Titan’s atmosphere.
The team also included other researchers at Berkeley Lab, the University of Hawaii at Manoa, Samara University in Russia, and Florida International University. The team used vacuum ultraviolet light experiments at Berkeley Lab’s Advanced Light Source (ALS), together with computer simulations and modeling work to demonstrate the chemical reactions that contribute to Titan’s modern-day atmospheric chemistry.
“We provide evidence here for a low-temperature reaction pathway that people have not thought about,” said Musahid Ahmed, a scientist in Berkeley Lab’s Chemical Sciences Division and co-leader of the study at the ALS. “This gives rise to a missing link in Titan’s chemistry.”
Titan may yield clues to the development of complex chemistry on other moons and planets, including Earth, he explained. “People use Titan to think about a ‘pre-biotic’ Earth – when nitrogen was more prevalent in the early Earth’s atmosphere.”
Benzene, a simple hydrocarbon with a six-carbon single-ring molecular structure, has been detected on Titan and is believed to be a building block for larger hydrocarbon molecules with two- and three-ring structures that, in turn, formed other hydrocarbons and aerosol particles that now make up Titan’s atmosphere. These multiple-ring hydrocarbon molecules are known as polycyclic aromatic hydrocarbons (PAHs).
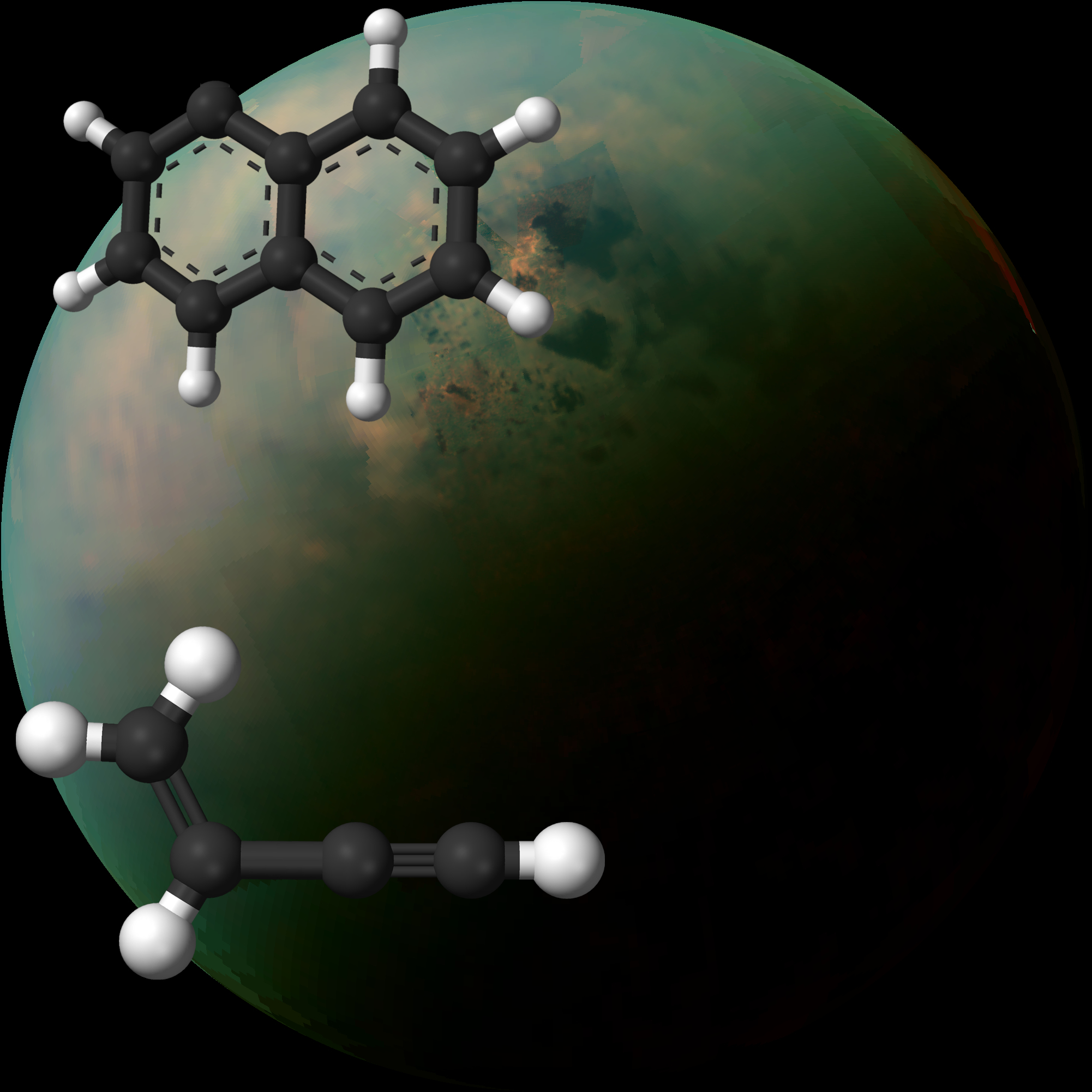
Scientists have explored the chemistry at work when combining two gases: one composed of two-ring molecular structure known as naphthyl radicals (upper left), and the other composed of a hydrocarbon called vinylacetylene (lower left). The white spheres represent hydrogen atoms and the dark spheres represent carbon atoms. Behind these 3-D molecular representations is an image of Saturn’s moon Titan, taken by NASA’s Cassini spacecraft. (Credits: Wikimedia Commons, NASA Jet Propulsion Laboratory, Caltech, Space Science Institute, John Hopkins University Applied Physics Laboratory, University of Arizona)
In the latest study, researchers mixed two gases – a short-lived two-ring PAH known as a naphthyl radical (C10H7) and a hydrocarbon called vinylacetylene (C4H4) – at the ALS, and produced three-ring PAHs in the process. Both of the chemicals used to drive the reaction are inferred to exist on Titan based on what is known about the chemical makeup of its atmosphere.
The ALS experiments jetted away the end products of the reactions from a small reaction chamber. Researchers used a detector known as a reflectron time-of-flight mass spectrometer to measure the mass of molecular fragments produced in the reaction of the two gases. Those measurements supplied details about the chemistry of the three-ring PAHs (phenanthrene and anthracene).
While the ALS experiments used a chemical reactor to simulate the chemical reaction and a beam of vacuum ultraviolet light to detect the products of the reaction, supporting calculations and simulations showed how the chemicals formed in the ALS experiments do not require high temperatures.
PAHs like the chemicals studied at the ALS have properties that make them particularly difficult to identify in deep space, Kaiser said. “In fact, not a single, individual PAH has been detected in the gas phase of the interstellar medium,” which is the material that fills the space between stars.
He added, “Our study demonstrates that PAHs are more widely spread than anticipated, since they do not require the high temperatures that are present around carbon stars. This mechanism we explored is predicted to be versatile and is expected to lead to the formation of even more complex PAHs.”
And because PAHs are considered as precursors to forming molecular clouds – the so-called “molecular factories” of more complex organic molecules that can include the precursors to life as we know it – “This could open up theories and new models of how carbon-containing material in deep space and in the rich atmospheres of planets and their moons in our solar system evolve and originate,” he said.
Alexander M. Mebel, a chemistry professor at Florida International University and co-leader of the study, carried out calculations that showed how the reactants can naturally come together and form new compounds at very low temperatures.
“Our calculations revealed the reaction mechanism,” Mebel said. “We showed that you don’t need any energy to drive the reaction of naphthyl and vinylacetylene, so the reaction should be efficient even in the low-temperature and low-pressure atmospheric conditions on Titan.”
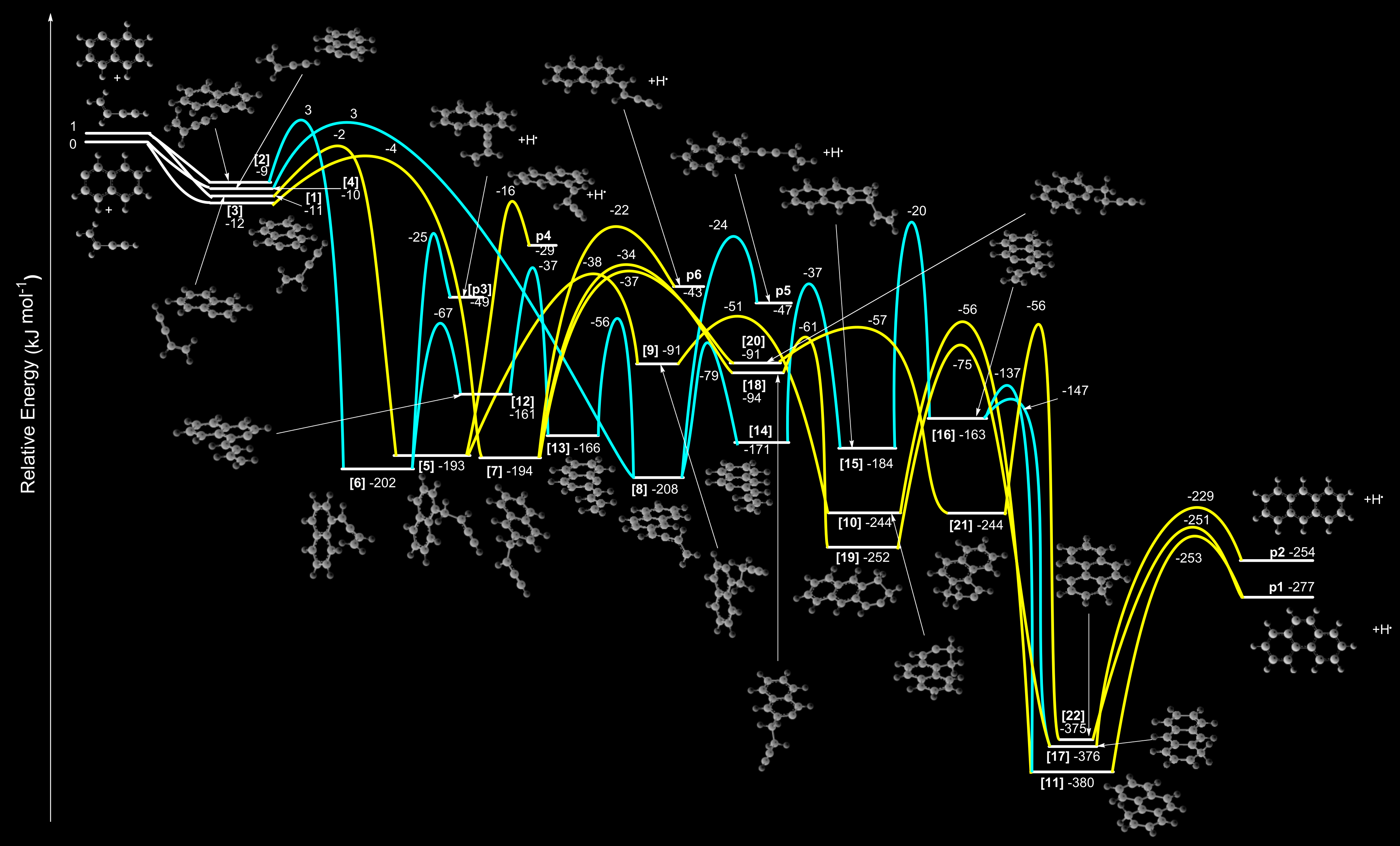
This chart shows calculations for potential energy surfaces in chemical reaction processes involving naphthyl radicals and vinylacetylene gases. The combination of these gases can produce a number of compounds, including three-ring molecular structures. (Credit: Long Zhao, Ralf I. Kaiser, et al., Nature Astronomy, DOI: 10.1038/s41550-018-0585-y)
A key to the study was in the detailed modeling of the reactor cell where the gases were mixed.
Mebel noted that modeling of the energies and simulations of the gas-flow dynamics in play within the reactor help to monitor reaction progress inside the reactor, and allowed researchers to tie theoretical results closely with experimental observations.
The modeling work, which helped to predict the chemicals produced in the reactions based on the initial gases and the temperature and pressure of the heated chamber where the gases were mixed and struck with the vacuum ultraviolet beam, was led by the research team at Samara University.
“This verification of the model, by comparing it with experiments, can also be helpful in predicting how the reaction would proceed in different conditions – from Titan’s atmosphere to combustion flames on Earth.”
An aim of the continuing research, Kaiser said, is to unravel the details of how carbon-containing compounds with similar structures to DNA and RNA can develop even in extreme environments.
The Advanced Light Source is a DOE Office of Science User Facility.
This work at Berkeley Lab, the University of Hawaii, and Florida International University was supported by the U.S. Department of Energy’s Office of Basic Energy Sciences, including the Division of Chemical Sciences, Geosciences, and Biosciences; modeling studies in Samara were sponsored by the Ministry of Education and Science of the Russian Federation.
# # #
Lawrence Berkeley National Laboratory addresses the world’s most urgent scientific challenges by advancing sustainable energy, protecting human health, creating new materials, and revealing the origin and fate of the universe. Founded in 1931, Berkeley Lab’s scientific expertise has been recognized with 13 Nobel Prizes. The University of California manages Berkeley Lab for the U.S. Department of Energy’s Office of Science. For more, visit www.lbl.gov.
DOE’s Office of Science is the single largest supporter of basic research in the physical sciences in the United States, and is working to address some of the most pressing challenges of our time. For more information, please visit http://science.energy.gov.