Contact: Dan Krotz, (510) 486-4019, [email protected]
Caged quantum dots explained. Scientists at Berkeley Lab
Soon, no cell will be able to keep its secrets. Berkeley Lab scientists have developed a nanosized crystal that lights up on command, a feat that could allow researchers to more easily observe individual proteins as they move inside cells.
The tiny beacon, which becomes luminescent after it’s zapped with ultraviolet light, can be attached to a protein and switched on when the protein does something worth spying on — like bind to a drug or move from one part of the cell to another.
The stealth probe could help scientists understand how some diseases interfere with the traffic patterns of cellular proteins, and more importantly, how to treat such diseases. It could also reveal how different parts of a cell change over time, protein by individual protein.
All thanks to a tiny crystal with a simple trick.
“When you hit it with light, it turns on, if you don’t, it remains dark,” says Bruce Cohen, a staff scientist in the Biological Nanostructures Facility at Berkeley Lab’s Molecular Foundry, a U.S. Department of Energy User Facility that provides support to nanoscience researchers around the world. Cohen and colleagues are working to bring the promise of nanotechnology — which harnesses the unique properties of particles that measure less than ten-billionths of a meter in diameter — to a wider audience, in this case biologists.
“We’re developing a way to track single molecules in living cells,” adds Cohen. “A cell is like a city. We want to know exactly where proteins within the cell live, and where they go.”
To do this, Cohen’s team turned to quantum dots, which are nanometer-sized crystals of semiconductors like cadmium selenide and cadmium sulfide. Paul Alivisatos, Berkeley Lab’s Deputy Director and Division Director of the Materials Sciences Division, developed a way to use quantum dots for bioimaging about ten years ago. Since then, quantum dots have proven themselves as highly effective luminescent labels for the study of living cells, among other applications. Unlike fluorescent labels derived from other compounds, they’re very bright and last a long time before fading.
Their only drawback is they’re always luminescent. This nonstop brightness is handy when scientists want to observe the goings-on throughout an entire cell. But biologists increasingly want to home in on a specific protein, in a specific part of a cell, at a specific time. To facilitate this, quantum dots need to be stifled until things get interesting. They need to be caged.
Cohen’s team created this cage by coating quantum dots with an organic molecule that inhibits the dots’ luminescence. To break the cage, they hit the dots with a pulse of ultraviolet light that is strong enough to sever a bond in the organic molecule, rendering it ineffective. Just like that, the dots are free to do what they do best: glow.
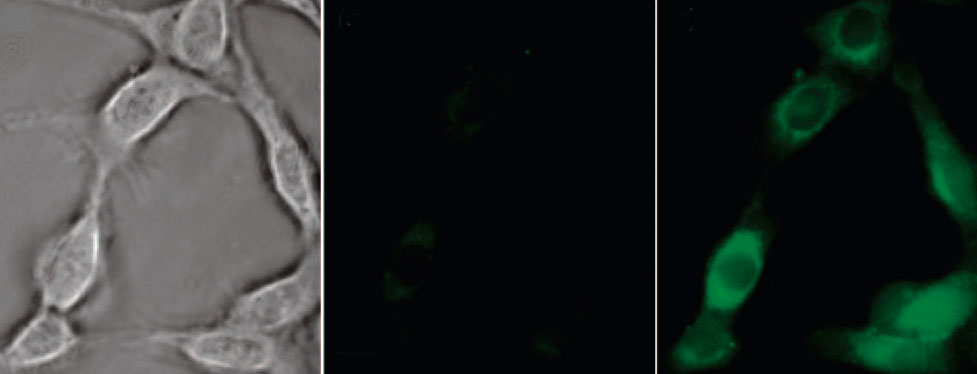
Quantum dots uncaged. These fibroblasts (left), which are cells found in the connective tissue of animals, have been incubated with caged quantum dots. The dots are dark at first (middle image), but are freed after they are hit with ultraviolet light (right).
In this way, caged quantum dots could usher in a new field of single protein studies. For example, biologists could use them to observe a class of cellular membrane proteins that are targeted by drugs such as heart medications and opiates such as morphine. What does each protein do when it binds with a pharmaceutical? Does it remain in the membrane, or does it sink into the cell’s interior?
“Answering these questions is important because the number of proteins in a cell membrane affects how sensitive it is to certain drugs,” says Cohen. “To understand disease and basic biology, it is critical to know how particles move around in a cell — and this technique can give scientists the ability to learn this information.”
The research is outlined in an article, “Caged Quantum Dots,” which was recently published online by the Journal of the American Chemical Society.
Additional Information:
- Learn more about Bruce Cohen’s research.
- Learn more about the Molecular Foundry.