Contact: Paul Preuss
Dark energy appears to account for over three-quarters of the stuff in the Universe, and it’s pushing all the rest – ordinary matter and dark matter – farther apart at an ever-increasing rate. But what is dark energy? Although theories abound, the short answer is that nobody knows.
We know it exists because of an experimental technique that uses specific types of exploding stars, or supernovae, as “standard candles.” A dozen years ago measurements of these supernova at increasing distances from Earth led to the unexpected discovery of dark energy; observations of supernovae continue to increase in power and precision in ongoing studies.
Independent evidence from measurements of the cosmic microwave background and other estimates of the matter density of the Universe provided early support for the radical idea of dark energy. Newer and quite different techniques, including weak lensing and baryon acoustic oscillations, are now poised to offer unique insights into what Nobel Prize-winner Frank Wilczek has called “the most fundamentally mysterious thing in basic science.”
Type Ia Supernovae: The Best Standard Candles
During the 1980s and 90s, the Supernova Cosmology Project (SCP), co-founded by Saul Perlmutter and Carl Pennypacker and based at Berkeley Lab, demonstrated that Type Ia supernovae were excellent standard candles for measuring the expansion history of the Universe. Although the idea had been circulating within the astronomical community for years, says Perlmutter, a Berkeley Lab astrophysicist and professor of physics at UC Berkeley, “In the early days, people thought measuring expansion with supernovae would be too hard.”
The SCP went on to show that distant supernovae, short-lived and unpredictable as they are, can nevertheless be collected “on demand,” allowing observers to schedule telescope time in advance and accumulate enough data to make confident estimates of expansion.
“In retrospect it seems obvious, but we realized that the whole process could be systematized,” Perlmutter explains. “By searching the same group of galaxies three weeks apart, we could find supernovae candidates that had appeared in the meantime. We could guarantee four to eight supernovae each time, and all of them would be on the way up” growing brighter instead of already fading.
Type Ia supernovae are among the brightest things in the Universe; what’s more, they are all almost the same brightness, with differences that can be standardized to less than 10 percent. Thus a supernova’s apparent brightness shows how far away it is and, because light takes time to travel, how far back in time it exploded.
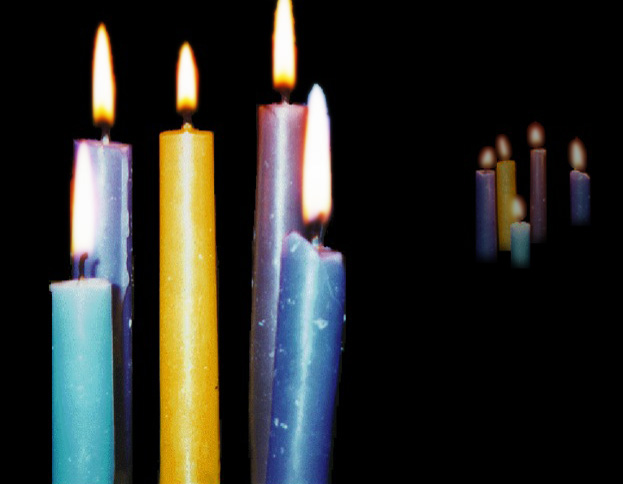
We can tell how far away a “standard candle” is by how bright or dim it appears. What goes for wax candles across the room goes for Type Ia supernovae across the Universe. (Image from Gerson Goldhaber)
The supernova’s redshift – the shifting of spectral lines (signals of specific elements in the exploding star) toward the red end of the spectrum – is a direct measure of how much the space through which the light has traveled has stretched.
The idea is simple on paper: by comparing brightness to redshift for numerous Type Ia supernovae, from nearby to very distant, an observer can tell how the rate of expansion of the Universe has changed over time.
Members of the Supernova Cosmology Project expected to find, as did their rivals in the High-Z Supernova Search Team, that the farther away (the farther back in time) a supernova was, the brighter (closer) it would appear relative to its redshift — an indication that expansion has been slowing. Instead both teams found the opposite.
“The chain of analysis was long, and the Universe can be devious, so at first we were reluctant to believe our result,” Perlmutter explains. “But the more we analyzed it, the more it wouldn’t go away.”
Perlmutter described the evidence for accelerating expansion at an American Astronomical Society meeting in January 1998. At first both teams thought the cause was a form of Einstein’s “cosmological constant,” assumed to be an unknown form of energy that uniformly, as its name suggests, counteracts the mutual gravitational attraction of the matter in the cosmos.
But within weeks a flurry of alternative explanations and theories were put forth, including ideas for a dynamical, not constant, form of energy, or for an odd cosmos in which our Universe bounces back and forth between expansion and contraction — or perhaps most radical of all, that Einstein’s General Theory of Relativity, the best explanation of gravitation we have, is flawed.
One way to sort out some of these competing theories is to collect a much larger sample of supernovae and measure them with greater precision. That way, scientists would be able to tell whether dark energy has indeed been constant and expansion has followed a smooth curve, or whether at different eras expansion has proceeded faster or slower than at present, and dark energy is dynamic.
To gather a lot more supernovae, especially more distant supernovae, it’s necessary for a telescope to escape the limitations of Earth’s atmosphere. In 1999, Berkeley Lab physicists and astronomers formed an international collaboration to design the SuperNova/Acceleration Probe (SNAP), a satellite dedicated to the study of dark energy. In 2003 the U.S. Department of Energy (DOE) and NASA formed the Joint Dark Energy Mission (JDEM) and solicited additional ideas. The DOE JDEM Project Office is located at Berkeley Lab.
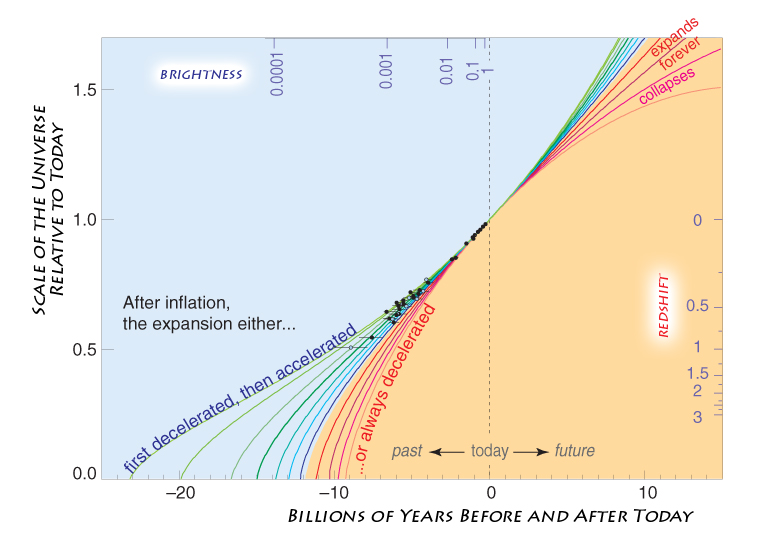
The brightness of Type Ia supernovae indicates their distance and therefore how long ago they exploded. Their redshift indicates how much the universe has expanded since then. Together, and with enough samples, their brightness and redshift indicate the expansion history of the Universe. Researchers expected to find that expansion was slowing, or at least coasting (red lines). Instead they found it accelerating (blue lines); expansion will probably continue to accelerate in the future. (Graphic from Saul Perlmutter in "Physics Today." Click on image for best resolution)
Better measurements of Type Ia supernovae require reducing or eliminating uncertainties in measuring their brightness and spectra. Brighter Type Ia supernovae wax and wane more slowly than fainter ones, for example, but when these individual “light curves” are stretched to fit the norm, and brightness is scaled according to the stretch, most can be made to match. This “classic” method has been used to standardize intrinsic brightness to within 8 to 10 percent.
To reduce these error bars and other uncertainties, more high-quality spectra are needed, beginning with “nearby” supernova, those whose spectra have not been shifted so far into the red that parts are hard to recover or no longer visible. Since its founding in 2002, the Nearby Supernova Factory (SNfactory), a collaboration of Berkeley Lab, a consortium of French laboratories, and Yale University, has amassed an enormous database of some 2,500 spectra.
With this data, SNfactory researcher Stephen Bailey found that simply by measuring the ratio of brightness between two specific regions in the spectrum of a Type Ia supernova taken on a single night, that supernova’s distance can be determined to better than 6 percent uncertainty.
Berkeley Lab cosmologist Greg Aldering, a founder and leader of the SNfactory, says, “This is an example of exactly what we designed the Nearby Supernova Factory to do. It underlines the vital role of detailed spectrometry in discoveries of cosmic significance.”
But supernovae alone cannot provide the whole answer. Baryon acoustic oscillation is a new technique that provides a “cosmic ruler” to measure the expansion history of the Universe. Read on>
Additional information
How dark energy was discovered is discussed in http://www.lbl.gov/Science-Articles/Archive/sabl/2007/Nov/darkenergy1.html (part 1); http://www.lbl.gov/Science-Articles/Archive/sabl/2007/Nov/darkenergy2.html (part 2); and http://www.lbl.gov/Science-Articles/Archive/sabl/2008/Feb/dark-energy.html (part 3).
How supernovae are used to measure dark energy is discussed in detail in http://www.lbl.gov/Science-Articles/Archive/sabl/2005/October/04-supernovae.html (part 1); http://www.lbl.gov/Science-Articles/Archive/sabl/2005/November/04-supernovae-2.html (part 2); and http://www.lbl.gov/Science-Articles/Archive/sabl/2006/Jan/05-supernovae-pt3.html (part 3).