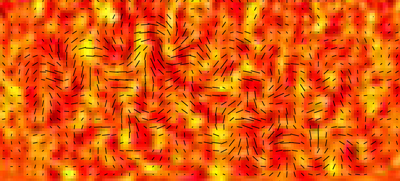
B-mode polarization of the cosmic microwave background radiation exhibits a distinctive 'curl,' either left or right-handed, imposed by the distortion of space as gravity waves moved through it. (Image BICEP2 Collaboration)
In 1996 Uros Seljak was a postdoc at Harvard, contemplating ways to extract information from the cosmic microwave background (CMB). The distribution of anisotropies, slight temperature differences, in the CMB had much to say about the large-scale structure of the universe. If it were also possible to detect the polarization of the CMB itself, however, a much wider window would be opened – polarization could even reveal the tracks of gravitational waves.
“Gravitational waves are distortions of space on a small scale, and have no consequence for the large-scale structure of the universe,” says Seljak, now a professor of physics and astronomy at UC Berkeley and a faculty scientist in Berkeley Lab’s Physics Division. “Both result from perturbations during inflation, but the seeds of large-scale structure are described by a scalar field, and gravitational waves by a tensor field.”
Cosmologists already assumed that inflation theory was essentially correct: virtually instantaneous expansion after the big bang explained how regions of the universe never otherwise in contact had in fact started from the same initial conditions. Here was a way to test inflation directly.
Seljak posted a paper to arXiv (soon published in the Astrophysical Journal), becoming the first to predict how polarization could be used to find CMB tensor signals, including gravitational waves. Marc Kamionkowski, Arthur Kosowsky, and Albert Stebbins of Fermilab independently posted their similar proposal shortly thereafter. In further work with Matias Zaldarriaga, Seljak named the E and B modes of CMB polarization, borrowing the symbols for light’s electric and magnetic fields – scalar fields produce E-mode polarization; a gravitational tensor field can produce both.
Fast forward to the year 2000, when Adrian Lee, a professor of astrophysics at UC Berkeley and faculty scientist in the Lab’s Physics Division, came up with the idea of suspending the recently invented transition-edge sensor bolometer (TES), creating what he describes as “a trampoline that heats up when an energy pulse lands on it” – the more energy, the bigger the reaction.
Lee integrated suspended TESs with other functions in flat chips suitable for the focal planes of CMB telescopes. He proposed just such a telescope, the POLARBEAR experiment, initiated with support from Berkeley Lab’s Laboratory Directed Research and Development (LDRD). BICEP2 and many other CMB telescopes also use versions of these focal-plane chips.
On Monday, March 17, 2014, the BICEP2 collaboration grabbed the brass ring: first detection of B-mode polarization from gravitational waves, thus first direct evidence of inflation – a signal far stronger than most scientists had expected.
“We look forward to working with BICEP2 to refine their measurements,” says Lee. “Until now, the best evidence for inflation was a slight ‘tilt’ in the CMB scalar field. POLARBEAR’s higher resolution could detect a similar tilt in the tensor field, a double confirmation of inflation.”
Among the many implications of the large B-mode signal, says Seljak: “It may force us in the direction of string theory. It also fits in with models of continuing inflation that produce multiple universes.”
Berkeley Lab has played an important part in opening the cosmic frontier to even wider vistas.
###
POLARBEAR data storage and processing are handled by the National Energy Research Scientific Computing Center (NERSC) through the Computational Cosmology Center (C3), which manages a huge amount of CMB data.