Media contact: Paul Preuss, (510) 486-6249
Scientific contact: Joel Ager, (510) 486-6715
Human bones are tough – even tougher to break in the transverse, crosswise direction than to split in the longitudinal, lengthwise direction. Although this trait has long been known, it has not been well understood, since most previous measurements were of cracks more than two or three millimeters in length, and they managed to detect only relatively small differences between bone toughness in the different orientations.
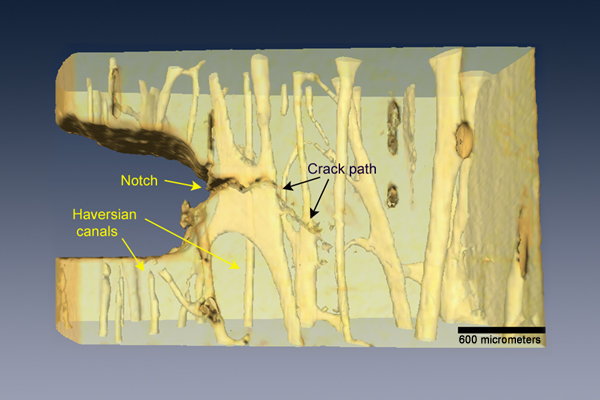
An x-ray tomograph from beamline 8.3.2 of the Advanced Light Source shows that bone is tougher in the transverse (crossways) orientation because a propagating crack must undergo marked deflections and twists as it interacts with the bone’s underlying structures.
Now, for the first time, a trio of scientists in Berkeley Lab’s Materials Sciences Division (MSD) have taken a close look at just how submillimeter cracks propagate through bone. Cracks of this length are far more common than longer ones, if less noticeable; indeed, whether or not a bone splits or breaks depends on how efficiently short cracks can be prevented from growing into longer ones. The researchers discovered that at the submillimeter scale, human bones are five times tougher in the transverse orientation than they are longitudinally, a far greater difference than previously thought.
“It has been known for a long time that bone is a tough material,” says Robert Ritchie, a faculty senior scientist in MSD and chair of UC Berkeley’s Materials Science and Engineering Department. “What we tried to do here is to make really accurate quantitative measurements of bone toughness in the presence of physiologically relevant small cracks – and to identify the origins of this toughness. What we found was that bone is actually far tougher than had been previously thought! Moreover, we were able to discern that the mechanisms associated with this toughening were quite different when bone splits compared to when it breaks transversely.”
Joel Ager, a staff scientist in MSD, says, “Bone is remarkably well designed on all scales, from the nanometer scale to the micrometer scale,” from features measured in billionths of a meter to those measured in millionths of a meter or more. Bone, a composite material, is strong beginning with its basic components, the nanometer-scale triple helices of collagen proteins combined with nanoscale mineral grains of calcium phosphate. Compound fibers and fiber bundles add strength, as do the micrometer-scale lamellae (layers of these fibers) that are deposited around osteons. Osteons are concentric rings of mineralized tissue, sheathed by cement layers and surrounding channels for blood vessels called Haversian canals; osteons are one of the basic structural units of bone.
Slowing cracks on the micrometer scale
To keep cracks from propagating too fast and too far, a growing crack may be deflected and twisted – for example, by the cement sheath of an osteon – or crack growth may be inhibited by proliferating microcracks in the region of a larger crack, or by bridging of uncracked material behind the crack tip, or even by the bridging of collagen fibers. Before the present study by Ritchie and Ager, led by postdoctoral researcher Kurt Koester, these mechanisms had mostly been studied for long cracks in the lengthwise direction.
To investigate short, transverse cracks, Koester made movies of sections of human arm bones (the humerus) in an environmental scanning electron microscope (ESEM), which allows study of water-containing samples. He cut rectangular sections of cortical bone – the hard, strong, load-bearing outer part of the bone – in both orientations. He carved a notch where a crack might begin propagating – the crack tip – and with the sample under the microscope, applied stress. What he found was a revelation.
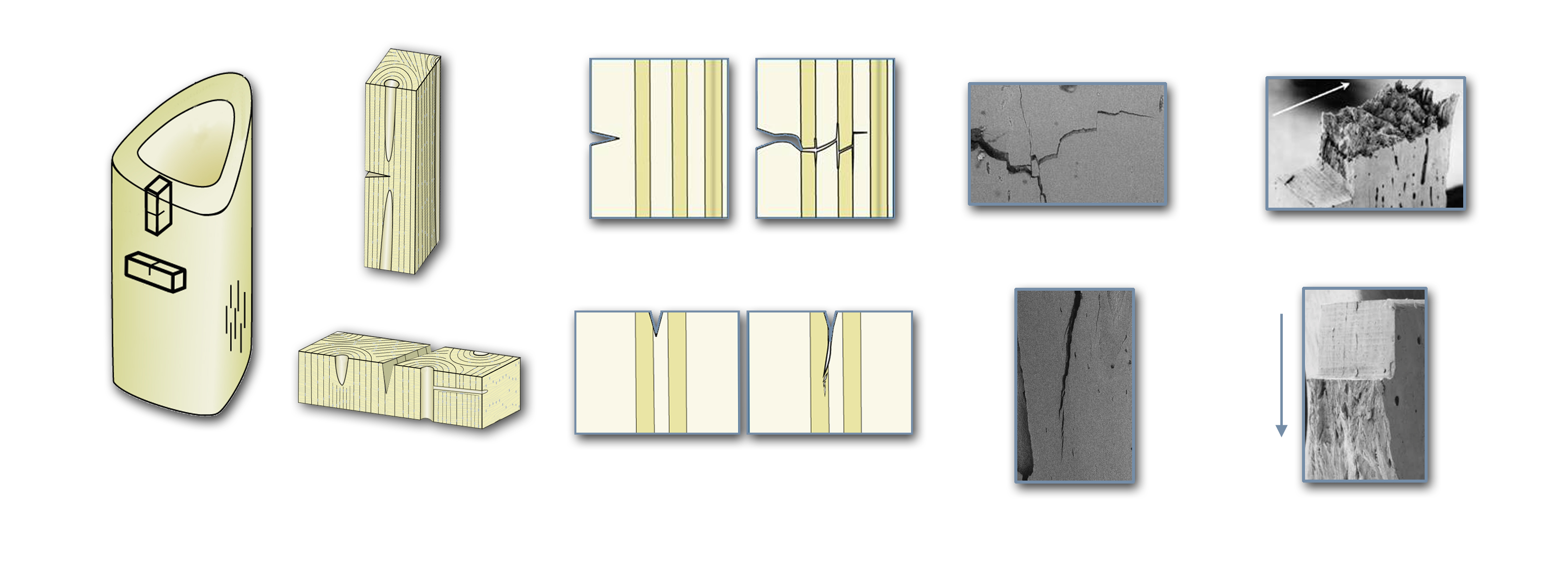
Samples from a human arm bone (humerus) were tested in an environmental scanning electron microscope, revealing the mechanisms which deflect and twist cracks in the transverse orientation (top), leading to five times greater toughness compared to the longitudinal orientation (bottom). For Kurt Koester’s movie of a propagating transverse crack, click this image.
Koester’s ESEM movies revealed the vital role played by osteons in increasing bone toughness. As a transverse crack begins to grow under increasing load, numerous microcracks are generated ahead of it; approaching the cement sheaths that surround the osteons, the microcracks align parallel to the sheath and perpendicular to the crack path. The effect is to deflect the crack longitudinally – rather than follow a straight path across the bone, a growing transverse crack zigzags, requiring far more force to drive it forward. (A similar phenomenon occurs on a smaller scale, at the interfaces between lamellae.)
Following these ESEM revelations of the role of microcracks, the researchers examined the same phenomena in three dimensions, using x-rays at the Advanced Light Source’s beamline 8.3.2 to generate tomographs of the zigzag paths of transverse cracks twisting and turning around osteons in a sample of cortical bone less than three millimeters across. In addition to deflection up and down the length of the bone, they discovered that the crack also twists sideways as it propagates, redirected by the orientation of the microcracks close to cement sheaths.
Says Koester, “In the transverse direction, the alignment of microcracks with the osteons gives rise to deflection and twisting. While microcracks are not a toughening mechanism in and of themselves, they give rise to the deflection and twisting of the propagating crack, which is a very potent toughening mechanism.”
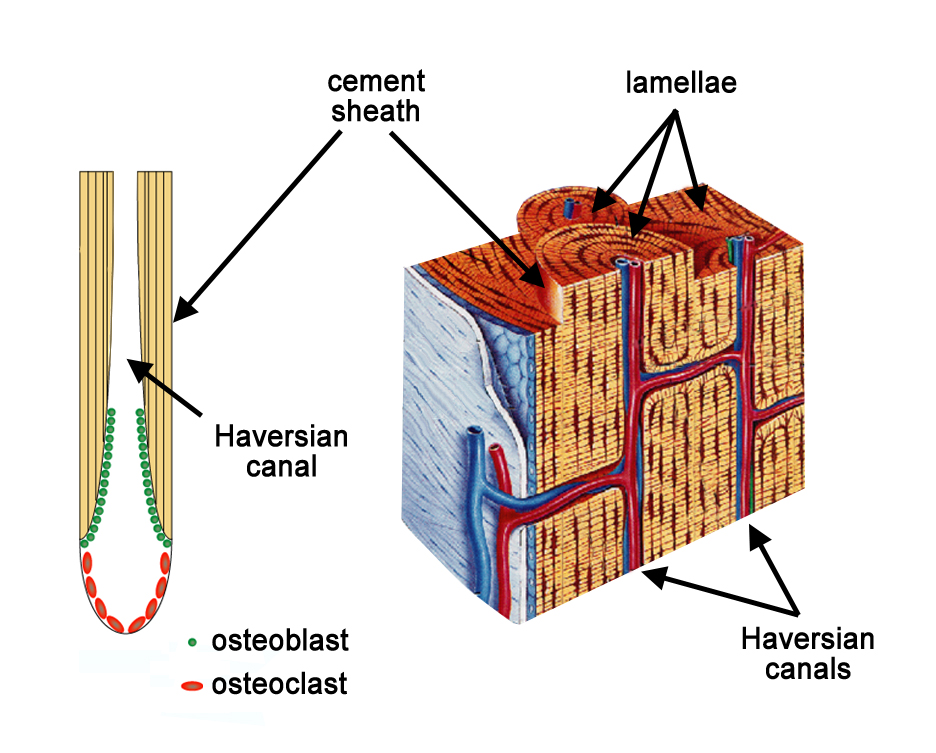
Bone remodeling constantly removes old bone and grows layers of new bone, forming osteons, the cement-sheathed basic units of bone structure, which surround Haversian canals carrying longitudinal blood vessels. Transverse canals are known as Volkmann’s canals.
In the longitudinal direction, cement sheaths are less likely to impede crack progression. Microcracks now run parallel with the growing crack; they still have a role to play, however, because by getting out ahead of the crack tip they allow the crack to bypass unbroken material, leaving bone “bridges” in the crack that resist further opening. Nevertheless, because of less deflection and twisting, cracks open more easily in the longitudinal direction – which is why bone splits more readily than it breaks.
Osteons are not simply structures; they are formed by a dynamic process of bone remodeling, which operates continually to remove (resorb) damaged bone and replace it with new growth. Almost like a tunneling machine, a vanguard of bone-eating cells called osteoclasts drills lengthwise through old, damaged bone, followed closely by cells called osteoblasts which lay down lamellae of new bone – first the outermost layers of the osteon, then subsequent inner layers, leaving a central opening, the Haversian canal, through which a blood vessel will run.
Because this process is ongoing, new osteons partially obliterate older osteons; over the first few decades of life a network of interspersed cement sheaths builds up, forming a dense barrier of deflectors in the path of transverse cracks.
As humans get older, however, bone toughness decreases. When bone remodeling malfunctions, by excessive resorption of old bone or inadequate replacement with new bone, or both, osteoporosis is the result. Yet many other factors are involved in bone fragility; to develop effective therapies for fragile bones, much more needs to be learned about how healthy bone manages to be so tough.
Additional information
“The true toughness of human cortical bone measured with realistically short cracks,” by Kurt Koester, Joel Ager, and Robert Ritchie, appears in the August, 2008 of Nature Materials and is available online to subscribers at http://www.nature.com/nmat/journal/v7/n8/full/nmat2221.html.
“Bone fracture: When the cracks begin to show,” by Peter Fratzl, appears in the same issue of Nature Materials and is available online to subscribers at http://www.nature.com/nmat/journal/v7/n8/full/nmat2240.html.
“Why human bone is more prone to fracture with age” discusses the mechanics of bone fragility. Visit http://www.lbl.gov/msd/PIs/Ritchie/04/04-11Ritchie_Bone.html.