Media contact: Dan Krotz, (510) 486-4019, [email protected]
Think Spring cleaning is difficult? Try cleaning up a toxic compound that is stubbornly moving through underground layers of clay and sand, interacting with microbes and minerals along the way. And, to make the job even more demanding, the cleanup effort must last thousands of years.
It’s one of the toughest jobs in environmental remediation — with only spotty success so far. That could change. Berkeley Lab scientists are pioneering a new approach to cleaning up underground contaminants that takes into account everything from the interactions of microscopic proteins to the characteristics of kilometer-scale field sites.
Specifically, Berkeley Lab’s Earth Sciences Division was recently awarded a $24.5 million, 5-year award from the Department of Energy’s Office Biological and Environmental Research to tackle the challenges that inhibit the long-term remediation of metals and radionuclides at several DOE sites, such as at the Hanford Site in Washington State and the Savannah River Site in South Carolina.
The results of their research will help scientists choose remediation strategies that are compatible with a site’s unique hydrological, biological, and geochemical conditions. It will also ensure the strategy works long after the last member of the cleanup crew leaves the site.
“We want to work with the system, rather than against it,” says Susan Hubbard, a staff scientist in the Earth Sciences Division and the research manager of the program, called a Sustainable Systems Scientific Focus Area. “We developed a five-year program that addresses the gaps in what we know about the remediation of metals and radionuclides. The ultimate goal is to develop insights about complex subsurface system behavior and associated tools that allow people to clean up contaminated sites in a sustainable way.”
Lessons learned from this wide-ranging effort could be applied to any subsurface area in need of a deep clean. The project will also inform other efforts, such as carbon sequestration, that require a broad understanding of subsurface processes in order to be successful.
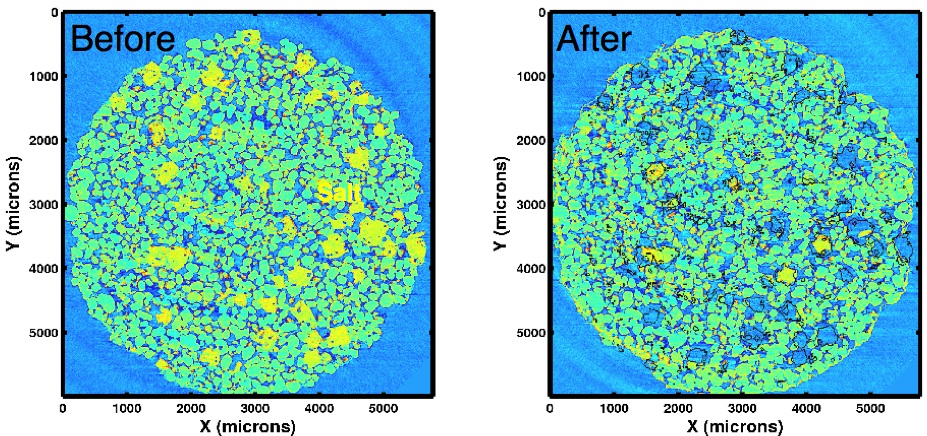
Time-lapse microtomography images obtained at the Advanced Light Source illustrate mineral dissolution, in this case salt crystals. Similar images could reveal the dissolution of calcites as acidic treatments are injected into the subsurface (image courtesy of Jonathan Ajo-Franklin of Berkeley Lab).
Hubbard adds that Berkeley Lab is well poised to head this effort because it boasts a range of expertise, from microbiologists and geophysicists to isotopic chemists and experts in reactive transport modeling.
“We are using all of our expertise to develop a predictive understanding of sustained environmental stewardship,” says Hubbard.
Berkeley Lab also has state-of-the-art tools that can be used to study the underground world in unprecedented detail. The Advanced Light Source is used to characterize how minerals, microbes, and contaminants react with each other, and the National Energy Research Scientific Computing Center is used to model how contaminants move through and react with various geological materials.
“The idea is to define the interactions of a subsurface system’s components, and even more importantly, learn how all of these components work together across a wide range of length and time scales,” says Hubbard.
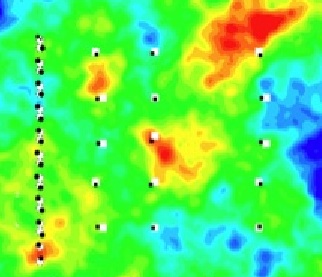
Computer simulation of biomass and iron sulfide precipitates in a uranium-contaminated aquifer in Rifle, Colorado (image courtesy of Li Li of Berkeley Lab).
Consider the complexity of a subsurface area the size of a two-bedroom house. It’s layered with clay and sand deposits, each of which has unique hydrological properties: water flows through sand more quickly than it does through clay. In addition, each layer has unique geochemical properties, meaning it reacts with different compounds in different ways.
Add to this scenario a toxic plume that must be remediated. No small feat, considering the plume moves through the sand and clay layers at different rates, interacting with adjacent minerals and microbial communities along the way. Suddenly, things get complicated. Next, add a remediation strategy, perhaps the subsurface injection of a slow-release polylactate that is designed to coax neighboring microbes into immobilizing the plume. It too changes the subsurface world. Now, things get very complicated.
To make sense of this spider’s web of inter-related processes, Hubbard and colleagues will mesh information gleaned from laboratory-scale biogeochemical research with field-scale observations of geophysical and hydrological processes. From this, they’ll develop remediation strategies that capture the complexity of a subsurface system.
“Over the last decade, we’ve come to realize that interactions between hydrological, geochemical, and microbiological properties significantly impact how a contaminant flows and reacts,” says Hubbard. “We need to understand how these properties control what we are doing over stewardship timeframes.”
Additional information:
Learn more about the Sustainable Systems Scientific Focus Area.