Forget the magnets on your fridge. How about a magnet in your fridge, one that keeps your leftover pizza cold while consuming less energy than today’s refrigerators?
It could happen. But don’t expect to buy an energy-saving magnetic fridge at Home Depot tomorrow, or even in five years. Scientists must first gain a better understanding of a phenomenon called the giant magnetocaloric effect, in which a changing magnetic field in a material causes its temperature to drop precipitously.
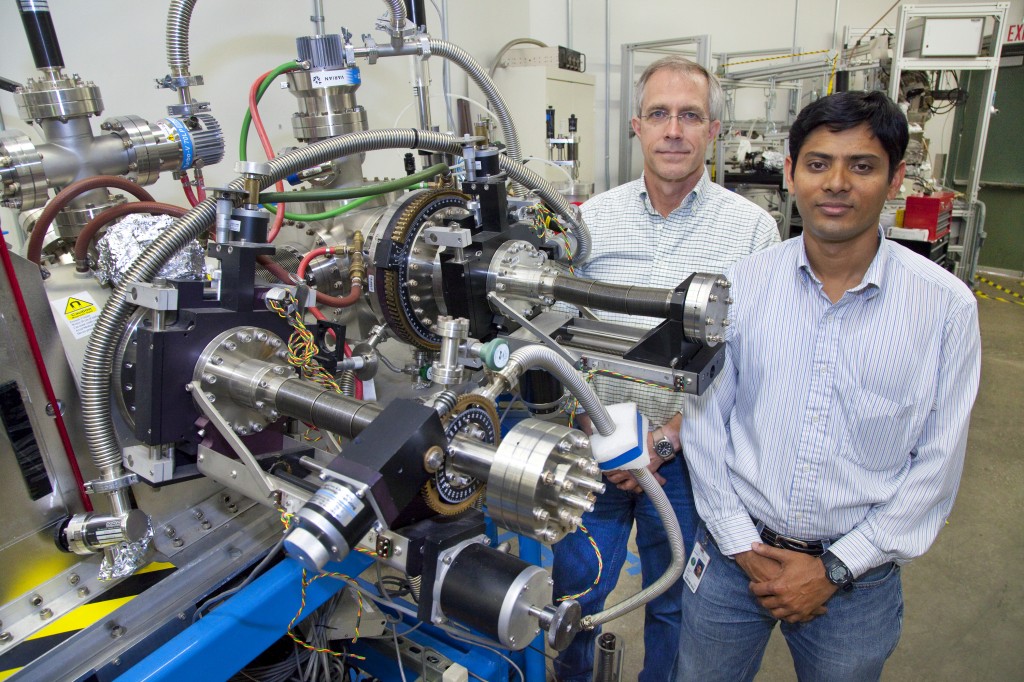
Fundamental research conducted at facilities like Berkeley Lab's Advanced Light Source could lead to the energy-saving technologies of tomorrow, such as a magnetic fridge. Jeff Kortright (left) and Sujoy Roy with an an endstation for soft x-ray resonant magnetic scattering and spectroscopy, which the scientists used at the Advanced Light Source's beamline 4.0.2 to learn more about the magnetocaloric effect in alloys. (Image by Roy Kaltschmidt, Berkeley Lab Public Affairs)
The idea is to use a material that exhibits this phenomenon as a refrigerant, like a high-tech block of ice.
“It’s a very promising concept. But to make it a reality, we first must learn in detail what’s happening inside materials as they undergo the giant magnetocaloric effect,” says Sujoy Roy, a physicist with Lawrence Berkeley National Laboratory.
Roy has spent the last few years uncovering the secrets of the phenomenon by studying alloys that experience a pronounced magnetocaloric effect. He uses Berkeley Lab’s Advanced Light Source, a national user facility that generates light brighter than the sun to probe the fundamental properties of substances, down to the atomic level.
Although the magnetocaloric effect was discovered more than 100 years ago, scientists have only recently eyed it as a way to develop solid-state refrigerators. A magnetic fridge would be quiet and compact. It wouldn’t require hydrofluorocarbons used in conventional refrigeration systems. Hydrofluorocarbons are potent greenhouse gases if they escape into the atmosphere. And a magnetic fridge would consume less electricity than today’s fridges, which account for eight percent of a family’s utility bill according to the U.S. Environmental Protection Agency.
The magnetocaloric effect could also cool laptops more efficiently than battery-draining fans, serve as the refrigerant in vehicle air conditioners, and be used in industrial refrigeration.
There’ve been successes over the years, but no big advances that put the magnetocaloric effect within reach of consumers. The trick is finding alloys that undergo the phenomenon under the conditions found in your kitchen or car. This means at room temperature and without requiring too much energy. It must also be affordable.
The hunt for ideal candidates has picked up speed over the past decade, with research taking place in the private sector as well as several universities and Department of Energy national laboratories. Much of the focus is on alloys that exhibit not just any magnetocaloric effect, but a “giant” magnetocaloric effect, so named because they exhibit a large entropy change.
“This is essential for practical applications,” Roy says. “These alloys are very interesting because they have the greatest potential to be used in refrigerators.”
Roy joined the pursuit in 2008 after reading a study conducted by Naushad Ali’s research group at Southern Illinois University. It described a nickel-manganese-gallium alloy in which copper was added. The alloy boasted some of the sought-after requirements: It had a huge magnetocaloric effect at room temperature. The scientists didn’t know why the alloy worked so well, however.
“So I contacted them and asked if I could help find out why,” Roy says.
They agreed, and within a few months Roy teamed up with Jeff Kortright of Berkeley Lab’s Materials Sciences Division and Elizabeth Blackburn of the United Kingdom’s University of Birmingham to study the alloy. They used x-ray magnetic circular dichroism and x-ray absorption spectroscopy at the Advanced Light Source to explore the changes in the local electronic and magnetic properties of each element in the alloy as its composition changes.
Among other findings, the team determined that the alloy’s magnetism weakens and its nickel-gallium bond strengthens when copper is added.
Their work doesn’t explain why nickel-manganese-gallium doped with copper boasts a huge magnetocaloric effect. But it fills in some blanks and offers one of the best looks yet of the phenomenon in action. Most importantly, research like this will help scientists zero in on alloys that are best suited to work in the real world, perhaps someday in your kitchen.
“If you know what is really happening in an alloy as it undergoes the magnetocaloric effect, then we can begin to think about adding other elements to get an even bigger effect – which is what we’re after,” says Roy. He hopes to soon expand his research and analyze alloys doped with lanthanide, iron, and silicon.
“We’re at a very important but early stage,” says Roy. “We have to understand the basic science surrounding the phenomenon before it can be used to develop useful technologies.”
More information:
- The scientists’ study, “Delocalization and hybridization enhance the magnetocaloric effect in Cu-doped Ni2MnGa,” was published in Physical Review B 79 235127 (2009).