Found in seashells, pearls, marble, and chalk, calcium carbonate is one of the most important molecules on Earth. It is also the most abundant form of carbon on our planet. But while scientists have studied calcium carbonate crystal growth for decades, they haven’t actually been able to explain how the crystals appear from the very start.
Now, researchers from Lawrence Berkeley National Laboratory (Berkeley Lab), the University of California Berkeley, and Pacific Northwest National Laboratory (PNNL) have used a high-powered electron microscope to capture the birth of these crystals, a phenomenon called nucleation. In doing so, they’ve demonstrated that pathways to different types of crystallization are varied and more complex than suspected.
It’s a first step, the researchers say, to better understanding how excess carbon dioxide might be pulled from the air and stored it in rock where it wouldn’t contribute to global warming.
“This work gives us insight into how nucleation and growth pathways happen in this difficult and complex chemical system,” explains Shaul Aloni staff scientist at Berkeley Lab’s Molecular Foundry, a U.S. Department of Energy User Facility. He says that designing chemical processes that could capture carbon dioxide from smoke stacks at power plants “starts with basic understanding of the formation of the mineral.”
Aloni worked with James De Yoreo of PNNL and formerly of the Molecular Foundry, and Michael Nielsen, of UC Berkeley. The work was published September 5 in the journal Science.
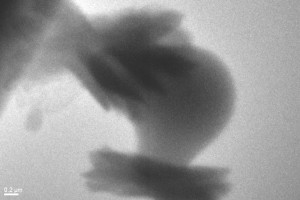
These crystals of aragonite, with the characteristic “sheaf of wheat” form, grew on the surface of a particle of amorphous calcium carbonate. Credit: Science
The process that transforms calcium and carbon atoms into crystals and eventually minerals starts with the formation of a small crystallite, a process called nucleation. But there has been no consensus about the mechanisms that govern it at the molecular level because nucleation is a spontaneous process where the initial crystal shape and structure is often different from the final one, making pathways to calcium carbonate difficult to study through direct observation.
To observe nucleation in real time, the researchers used a novel experimental setup allowing them to see inside an ultra-thin liquid layer where the reaction occurs. They mixed the calcium and carbon in liquid form inside small, custom-made sample holders fabricated by Hummingbird Scientific. And they captured the evolution of the crystals’ shapes and forms using a high-resolution transmission electron microscope at the Molecular Foundry.
Their results, summarized in video and still images, show the complexity of the reactions, particle by particle, and identify the transformations crystalline structures as they grow. All the forms of calcium carbonate nucleated and grew into crystals that look like balls, straight-edged diamonds, and sheaves of wheat, shapes that are associated with well-known variations of calcium carbonate.
Many different formations were sometimes observed in a single experiment. Moreover some crystals formed through a two-step process. For example, ball-shaped amorphous molecules of calcium carbonate nucleated first, then small crystals of the wheat-shaped aragonite formation (see video) or another kind of formation called vaterite appeared on their surfaces. These crystals continued to grow by consuming the calcium carbonate from the ball shaped particles.
Video: Amorphous calcium carbonate (round particle) forms and grows in size, then transforms into aragonite, which looks like bundles of wheat. The aragonite consumes almost the whole particle of amorphous calcium carbonate.
“It’s actually very important from a materials point-of-view that all the phases of calcium carbonate can form at the same time or transition from one form to the other,” Aloni says. Calcium carbonate’s formation is somewhat controversial, he adds, as no one knew which phases are favored in the crystallization process. While the current research doesn’t answer all the questions, it does confirm that many phases do in fact co-exist and that the calcium carbonate nucleation and growth processes are far more complex then previously thought.
By investigating these fundamental questions, researchers can begin to consider processes that could eventually convert carbon dioxide in the air into calcium carbonate inside rock. Toward that end, the team plans to explore various ways that that nucleation of calcium carbonate can be controlled when they alter the environment in future experiments. “We’re going to try to control the whole process,” Aloni says, “and introduce templates that can steer the crystal growth in the desired direction. ”
This work was supported by the DOE Office of Science.
For more information, read the PNNL news release here.