VIDEO: Simulation of self-assembling 2D nanocrystal/MOF superstructure. (Credit: Jeff Urban et al./Berkeley Lab)
Out of the box, MOFs (metal-organic frameworks) look like ordinary salt crystals. But MOFs are anything but ordinary – deep within each crystalline “grain” lies an intricate network of thin, molecular cages that can pull harmful gas emissions like carbon dioxide from the air, and contain them for a really long time.
But what if you could design a dual-purpose MOF material that could store carbon dioxide gas molecules for now, and turn them into useful chemicals and fuels for later? Researchers at the U.S. Department of Energy’s Lawrence Berkeley National Laboratory (Berkeley Lab) have devised a way to do just that – through a self-assembling “superstructure” made of MOFs and nanocrystals.
The study, which suggests that the self-assembling material has potential use in the renewable energy industry, was published in the journal Angewandte Chemie.
When ‘oil and water’ don’t mix
For years, researchers have tried to combine catalytic nanocrystals and crystalline MOFs into a hybrid material, but conventional methods don’t provide effective strategies for combining these two contrasting forms of matter into one material.
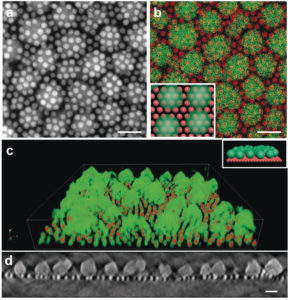
TEM images of a self‐assembled nanocrystal‐MOF superstructure. Berkeley Lab researchers discovered that iron-oxide nanocrystals and MOFs self-assemble into a ‘sesame-seed ball’ configuration. (Credit: Jeff Urban et al./Berkeley Lab
For example, one popular method known as X-ray lithography doesn’t work well with MOFs because these porous materials can be easily damaged by an X-ray beam and are challenging to manipulate, said Jeff Urban, the study’s lead author and facility director of Inorganic Nanostructures at Berkeley Lab’s Molecular Foundry, a DOE Office of Science User Facility specializing in nanoscience research.
The other problem is that although MOFs and nanocrystals can be mixed in a solution, researchers who have attempted to use methods of self-assembly to combine them have not been able to overcome the natural tendency of these materials to eventually move away from each other – much like the separation you see a few minutes after mixing a homemade salad dressing made of olive oil and vinegar.
“Metaphorically, the dense nanocrystal ‘billiard ball’ goes to the bottom, and the less-dense MOF ‘sponge’ floats to the top,” said Urban.
Creating a MOF-nanocrystal material that doesn’t separate as oil and water do after being mixed together requires “exquisite control over surface energies, often outside the reach of contemporary synthetic methods,” Urban said.
And because they’re not partnering well, MOFs (the material enabling long-term storage and separation) can’t sit next to nanocrystals (the material providing short-term binding and catalysis).
“For applications like catalysis and energy storage, there are strong scientific reasons for combining more than one material,” he added. “We wanted to figure out how to architect matter so you have MOFs and catalytic nanocrystals next to one another in a predictable way.”
How opposites attract through thermodynamics
So Urban and his team turned to the power of thermodynamics – a branch of physics that can guide scientists on how to join two materials with two completely different functions, such as energy storage versus catalysis/chemical conversion – into a hybrid superstructure.
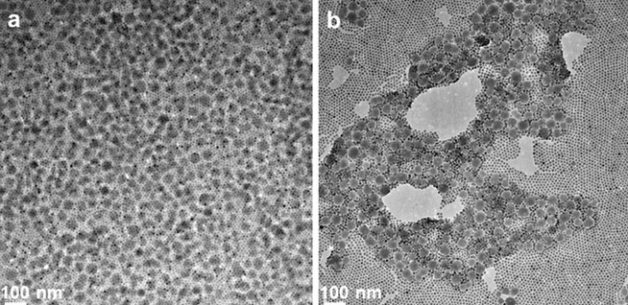
TEM images of MOFs and iron-oxide nanocrystals self-assembling into a bilayer film. (Credit: Jeff Urban et al./Berkeley Lab)
Based on their thermodynamics-based calculations, led by Steve Whitelam, a staff scientist at the Molecular Foundry, the Berkeley Lab researchers predicted that the MOF nanoparticles would form a top layer through molecular bonds between the MOFs and nanocrystals.
Their simulations, carried out at the National Energy Research Scientific Computing Center (NERSC) – another DOE Office of Science User Facility at Berkeley Lab – also suggested that a formulation of iron-oxide nanocrystals and MOFs would provide the structural uniformity needed to direct the self-assembly process, Urban said.
“Before we started this project a few years ago, there weren’t any real guiding principles on how to make MOF-nanocrystal superstructures that would hold up for practical, industrial applications,” Urban said. “These calculations ultimately informed the experiments used to fine-tune the energetics of the self-assembly process. We had enough data predicting that it would work.”
A crystal-clear image with a surprising result
After many rounds of testing different formulations of nanocrystal-MOF molecular bonds, STEM (scanning transmission electron microscopy) images taken at the Molecular Foundry’s National Center for Electron Microscopy (NCEM) confirmed that the MOFs self-assembled with the iron-oxide nanocrystals in a uniform pattern.
The researchers then used a technique known as resonant soft X-ray scattering (RSoXS) at the Advanced Light Source – a DOE Office of Science User Facility that specializes in lower energy, “soft” X-ray light for studying the properties of materials – to confirm the structural order observed in the electron microscopy experiments.
What they saw next surprised them.
“We expected the iron-oxide nanocrystals and MOFs to self-assemble, but we weren’t expecting the ‘sesame-seed ball’ configuration,” Urban said, referring to a fried Chinese pastry.
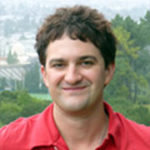
A research team led by Berkeley Lab’s Jeff Urban of the Molecular Foundry has designed a self-assembling MOF-nanocrystal material that could one day be used to turn CO2 emissions into renewable fuels. (Credit: Berkeley Lab)
In the field of self-assembly, scientists usually expect to see a 2D lattice. “This configuration was so unexpected. It was fascinating – we weren’t aware of any precedent for this phenomenon, but we had to find out why this was occurring.”
Urban said that the sesame-seed ball configuration is formed by a reaction between the materials that minimizes the thermodynamic self-energy of the MOF with the self-energy of the iron-oxide nanocrystal. Unlike previous MOF/nanocrystal interactions, the molecular interactions between the MOF and the iron-oxide nanocrystal drive the self-assembly of the two materials without compromising their function.
The new design is also the first to loosen rigid requirements for uniform particle sizes of previous self-assembly methods, opening the door for a new MOF design playbook for electronics, optics, catalysis, and biomedicine.
Now that they’ve successfully demonstrated the self-assembly of MOFs with catalytic nanocrystals, Urban and his team hope to further customize these superstructures using material combinations targeted for solar energy storage applications, where waste chemicals could be turned into feedstocks for renewable fuels.
Co-authors with Urban are Molecular Foundry researchers Steve Whitelam and Zdenek Preisler, who led calculations at NERSC; Peter Ercius, Karen Bustillo, and Chengyu Song, who contributed to the microscopy work at NCEM; Fen Qiu, who led experiments at the Molecular Foundry; and Michael Brady, formerly of the Advanced Light Source.
###
Lawrence Berkeley National Laboratory addresses the world’s most urgent scientific challenges by advancing sustainable energy, protecting human health, creating new materials, and revealing the origin and fate of the universe. Founded in 1931, Berkeley Lab’s scientific expertise has been recognized with 13 Nobel Prizes. The University of California manages Berkeley Lab for the U.S. Department of Energy’s Office of Science. For more, visit http://www.lbl.gov.
DOE’s Office of Science is the single largest supporter of basic research in the physical sciences in the United States, and is working to address some of the most pressing challenges of our time. For more information, please visit the Office of Science website at science.energy.gov.