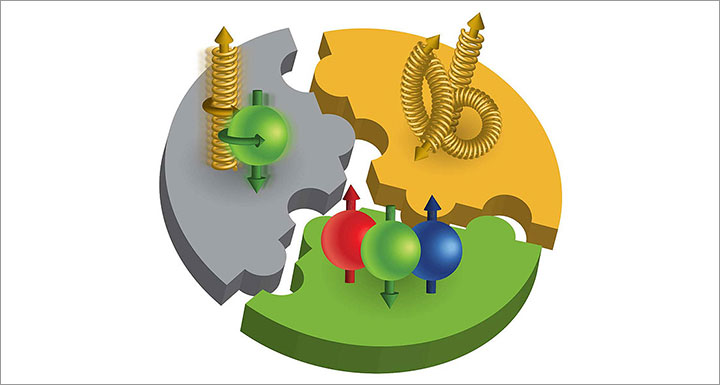
The proton spin puzzle: Scientists want to know how different constituents of the proton contribute to its spin, a fundamental property that plays a role in how these building blocks give rise to nearly all visible matter in the universe. Pieces of the puzzle include the orbital angular momentum of quarks and gluons (top left), gluon spin (top right) and quark and antiquark spin (bottom). The latest data from RHIC reveal that the antiquarks’ contribution is more complex than previously thought. (Credit: Brookhaven National Laboratory)
Note: This press release is adapted from a release produced by Brookhaven National Laboratory. View the original release.
New data from the STAR experiment at the Relativistic Heavy Ion Collider (RHIC) add detail – and complexity – to an intriguing puzzle that scientists have been seeking to solve: how the building blocks that make up a proton contribute to its spin. The results, published as a rapid communication in the journal Physical Review D, reveal definitively for the first time that different “flavors” of antiquarks contribute differently to the proton’s overall spin – and in a way that’s opposite to those flavors’ relative abundance.
Researchers at the Department of Energy’s Lawrence Berkeley National Laboratory (Berkeley Lab) had important roles in the latest results.
“This measurement shows that the quark piece of the proton spin puzzle is made of several pieces,” said James Drachenberg, a deputy spokesperson for STAR from Abilene Christian University. “It’s not a boring puzzle; it’s not evenly divided. There’s a more complicated picture and this result is giving us the first glimpse of what that picture looks like.”
It’s not the first time that scientists’ view of proton spin has changed. There was a full-blown spin “crisis” in the 1980s when an experiment at the European Center for Nuclear Research (CERN) revealed that the sum of quark and antiquark spins within a proton could account for, at best, a quarter of the overall spin. RHIC, a U.S. Department of Energy Office of Science user facility for nuclear physics research at Brookhaven National Laboratory, was built in part so scientists could measure the contributions of other components, including antiquarks and gluons (which “glue” together, or bind, the quarks and antiquarks to form particles such as protons and neutrons).
Antiquarks have only a fleeting existence. They form as quark-antiquark pairs when gluons split.
“We call these pairs the quark sea,” Drachenberg said. “At any given instant, you have quarks, gluons, and a sea of quark-antiquark pairs that contribute in some way to the description of the proton. We understand the role these sea quarks play in some respects, but not in respect to spin.”
Exploring flavor in the sea
One key consideration is whether different “flavors” of sea quarks contribute to spin differently.
Quarks come in six flavors – the up and down varieties that make up the protons and neutrons of ordinary visible matter, and four other more exotic species. Splitting gluons can produce up quark/antiquark pairs, down quark/antiquark pairs – and sometimes even more exotic quark/antiquark pairs.
“There is no reason why a gluon would prefer to split into one or the other of these flavors,” said Ernst Sichtermann, a STAR collaborator at Berkeley Lab who played a lead role in the sea quark research. “We’d expect equal numbers (of up and down pairs), but that’s not what we are seeing.” Measurements at CERN and DOE’s Fermi National Accelerator Laboratory have consistently found more down antiquarks than up antiquarks.
“Because there is this surprise – an asymmetry in the abundance of these two flavors – we thought there might also be a surprise in their role in spin,” Drachenberg said. Indeed, earlier results from RHIC indicated there might be a difference in how the two flavors contribute to spin, encouraging the STAR team to do more experiments.
Jinlong Zhang, as a postdoctoral researcher in Sichtermann’s research group at Berkeley Lab, played a lead role in the data analysis for this work, and was first to release the preliminary results for the STAR collaboration at the latest International Nuclear Physics Conference. Zhang’s analysis benefited from use of the Cori supercomputer at Berkeley Lab’s National Energy Research Scientific Computing Center (NERSC). Zhang is now at Stony Brook University.
“This is simultaneously a beautiful and challenging measurement,” said Feng Yuan, who leads the Nuclear Theory Program at Berkeley Lab.
Yuan, who didn’t participate in the latest study but is familiar with the work, added, “Nucleon spin physics has surprised us many times in the last three decades. This latest result from the STAR measurement of sea quark polarization adds an intriguing twist to this puzzle. It will certainly stimulate further experimental exploration, particularly at the planned electron-ion collider. I congratulate my colleagues at Berkeley Lab who have made significant contributions towards this analysis, and to the STAR collaboration as well.”
Delivering on spin goals
This result represents the accumulation of data from the 20-year RHIC spin program. It is the final result from one of the two initial pillars motivating the spin program at the dawn of RHIC.
For all of these experiments, STAR analyzed the results of polarized proton collisions at RHIC – collisions where the overall spin direction of RHIC’s two beams of protons was aligned in particular ways. Looking for differences in the number of certain particles produced when the spin direction of one polarized proton beam is flipped can be used to track the spin alignment of various constituents – and therefore their contributions to overall proton spin.
For the sea quark measurements, STAR physicists counted electrons and positrons – antimatter versions of electrons that are the same in every way except that they carry a positive rather than a negative electric charge. The electrons and positrons come from the decay of particles called W bosons, which also come in negative and positive varieties, depending on whether they contain an up or down antiquark.
The difference in the number of electrons produced when the colliding proton’s spin direction is flipped indicates a difference in W- production and serves as a stand in for measuring the spin alignment of the up antiquarks. Similarly, the difference in positrons comes from a difference in W+ production and serves the stand-in role for measuring the spin contribution of down antiquarks.
New detector, added precision
The latest data include signals captured by STAR’s endcap calorimeter, which picks up particles traveling close to the beamline forward and rearward from each collision. With this new data added to data from particles emerging perpendicular to the collision zone the scientists have narrowed the uncertainty in their results. The data show definitively, for the first time, that the spins of up antiquarks make a greater contribution to overall proton spin than the spins of down antiquarks.
“This ‘flavor asymmetry,’ as scientists call it, is surprising in itself, but even more so considering there are more down antiquarks than up antiquarks,” said Qinghua Xu of Shandong University, another lead scientist who supervised one of the graduate students whose analysis was essential to the paper. Xu is also a former member of the Berkeley Lab research team.
As Sichtermann noted, “If you go back to the original proton spin puzzle, where we learned that the sum of the quark and antiquark spins accounts for just a fraction of proton spin, the next questions are what is the gluon contribution? What is the contribution from the orbital motion of the quarks and gluons? But also, why is the quark contribution so small? Is it because of a cancellation between quark and antiquark spin contributions? Or is it because of differences between different quark flavors?
“Previous RHIC results have shown that gluons play a significant role in proton spin. This new analysis gives a clear indication that the sea also plays a significant role. It is far more complicated than just gluons splitting into any flavor you like – and a very good reason to look deeper into the sea.”
Bernd Surrow, a physicist from Temple University who helped develop the W boson method and supervised two of the graduate students whose analyses led to the new publication, agrees. “After multiple years of experimental work at RHIC, this exciting new result provides a substantially deeper understanding of the quantum fluctuations of quarks and gluons inside the proton. These are the kinds of fundamental questions that attract young minds – the students who will continue to expand the limits of our knowledge.”
Additional STAR measurements might offer insight into the spin contributions of exotic quark/antiquark pairs. In addition, U.S. scientists hope to delve deeper into the spin mystery at a proposed future Electron-Ion Collider. This particle accelerator would use electrons to directly probe the spin structure of the internal components of a proton – and should ultimately solve the proton spin puzzle.
Research at RHIC is funded primarily by the DOE Office of Science (NP). The STAR endcap calorimeter used for the forward and rearward measurements included in this analysis was funded in large part by the National Science Foundation. It was built at Indiana University under the leadership of STAR collaborator Will Jacobs.
Graduate Devika Gunarathne sand Amani Kraishan of Temple University (Kraishan is now at Al-Hussein Bin Talal University in Jordan) also participated in the data analysis for the STAR results.
RHIC and NERSC are DOE Office of Science User Facilities.
###
Founded in 1931 on the belief that the biggest scientific challenges are best addressed by teams, Lawrence Berkeley National Laboratory and its scientists have been recognized with 13 Nobel Prizes. Today, Berkeley Lab researchers develop sustainable energy and environmental solutions, create useful new materials, advance the frontiers of computing, and probe the mysteries of life, matter, and the universe. Scientists from around the world rely on the Lab’s facilities for their own discovery science. Berkeley Lab is a multiprogram national laboratory, managed by the University of California for the U.S. Department of Energy’s Office of Science.
Brookhaven National Laboratory is supported by the Office of Science of the U.S. Department of Energy. The Office of Science is the single largest supporter of basic research in the physical sciences in the United States, and is working to address some of the most pressing challenges of our time. For more information, please visit science.energy.gov.