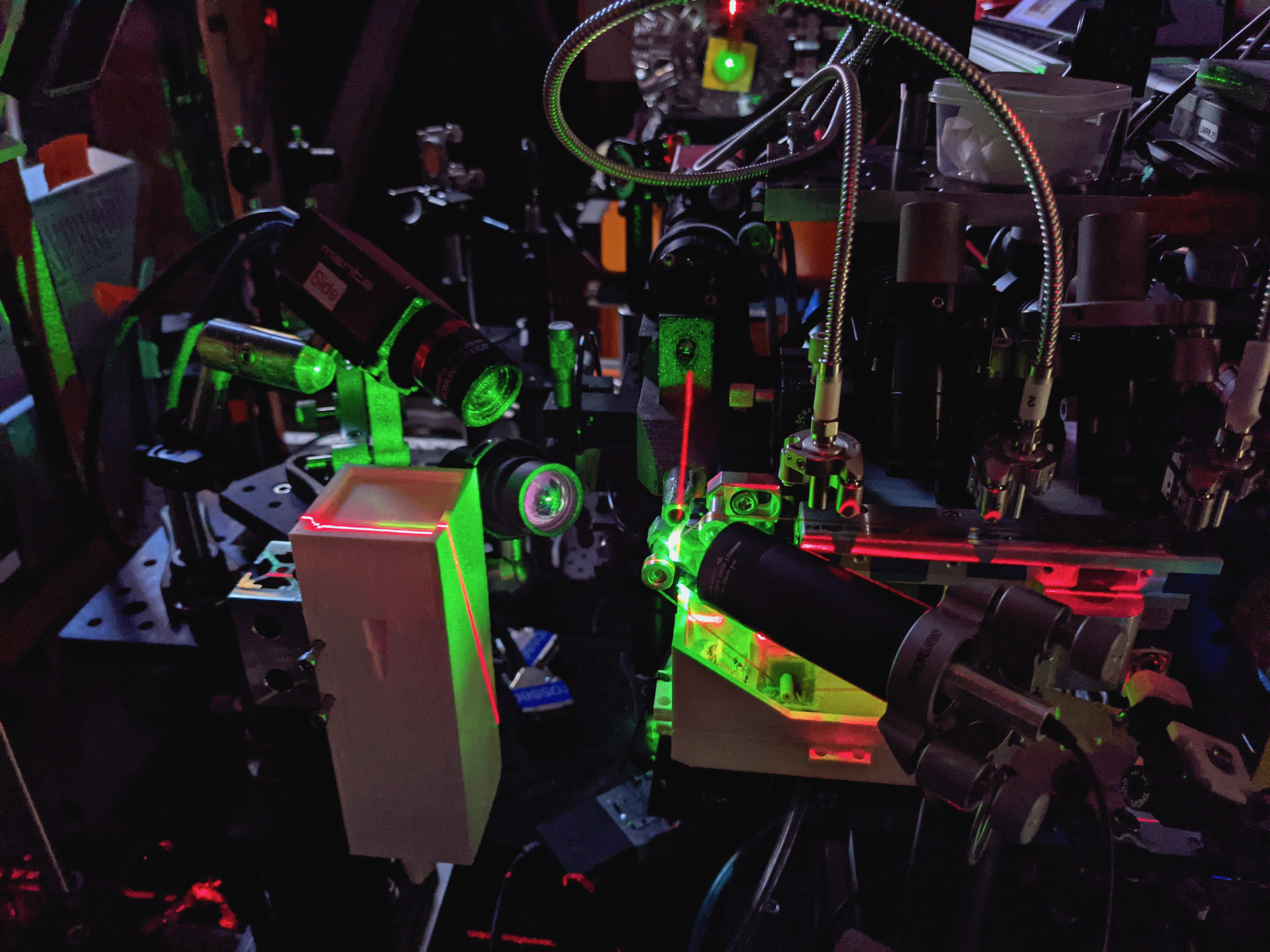
A photo of the XFEL equipment during laser alignment. The three metal-covered fibers (the chainmail-like tubes in top right corner) illuminate the protein samples and cycle them through part of the photosynthetic reaction. The green point in the center is the interaction point where the XFEL will hit the samples. (Credit: Hiroki Makita/Berkeley Lab)
Berkeley Lab scientists specialize in investigating fundamental scientific questions that, when answered, could lead to world-changing advances in technology, medicine, or energy.
One of these big questions is how, exactly, photosynthesis occurs. The enzyme-based process of converting carbon dioxide into food, using water and sunlight, is literally the foundation of life on Earth – and understanding the reaction at an atomic level could lead to vast production of renewable fuels made from greenhouse gases sucked out of the air. Pretty world changing, indeed.
A team from Berkeley Lab’s Molecular Biophysics and Integrated Bioimaging (MBIB) Division has been uncovering precise, step-by-step details of photosynthesis for years. They have just revealed another aspect, which was published earlier this month in Nature Communications. Their current work focuses on photosystem II (PS II), the enzyme that splits water into oxygen gas, hydrogen ions, and the free electrons that power the rest of the process, ultimately creating sugar molecules.
We spoke to two members, co-lead author and senior scientist Vittal Yachandra and co-first author and postdoctoral researcher Philipp Simon, about their latest milestones, shooting stuff with lasers, and why they chose this field.
Q. What did you discover in your latest paper?
Yachandra: The splitting of water using sunlight by the protein PS II, which is present in all plants
embedded in a membrane within chloroplasts called the thylakoid membrane, generates all the oxygen that we breathe. Interestingly, water, which is a ubiquitous “solvent” for all biological processes, is also a “substrate” for this reaction, meaning it is one of the reactants for this enzyme. That raises the question of how water is funneled into the active site – the area of the enzyme where the action happens – of PS II, which contains a metallic manganese-calcium complex (Mn4Ca).
This may be an important aspect of the active site, to prevent water from interacting with it prematurely, resulting in unwanted and deleterious intermediates such as peroxide from forming, which can cause damage to the protein.
We also found that PS II’s [known] proton channel actually also contains a “proton gate.” Protons are generated during the water-splitting
reaction, and they need to be removed from the catalytic site. This gate essentially prevents the proton from coming back to the catalyst and makes it a one-way street.
These two discoveries show how important the whole protein, not just the metal site, is for carrying out the catalytic reaction. And figuring out how PS II carries out the water-splitting reaction is an important part of our research and one of the grand science challenges to the Department of Energy.
Q. What is an X-ray free electron laser and why did you use it?
Yachandra: X-ray free electron laser (XFEL) is a tool developed for generating very intense, ultra-short pulses of X-rays. These X-ray laser pulses allow us to understand the properties of matter at the scale of atoms and molecules, and in the time scale of atomic motions and chemical reactions. XFEL systems point a super-focused photon beam (with a wavelength the size of an atom) at a sample of molecules and takes snapshots of how the photons diffract off the molecule. The entire process, from pulse to image, is completed in a few quadrillionths of a second.
Simon: There are two main advantages I’d like to mention: the first is that it uses an ultra-short X-ray pulse to get images that are then interpreted to map structures. Intense X-rays, like those used in traditional crystallography, damage and ultimately destroy proteins, especially the metallic catalytic centers. But XFELs allow us to probe our structures faster than the damage is caused, so it can “outrun it.” The other advantage is our structures are determined under very well-controlled illumination conditions and at room temperature. Imagine you want to study the flow of water, but it’s a cold (non-Californian) winter and all is frozen; it’s different, right? The same holds true for proteins, especially in this work focusing on water dynamics and the functions of amino acids. We want them to be able to move and react as they would in nature, only at room temperature we can understand how the protein orchestrates the catalytic reaction.
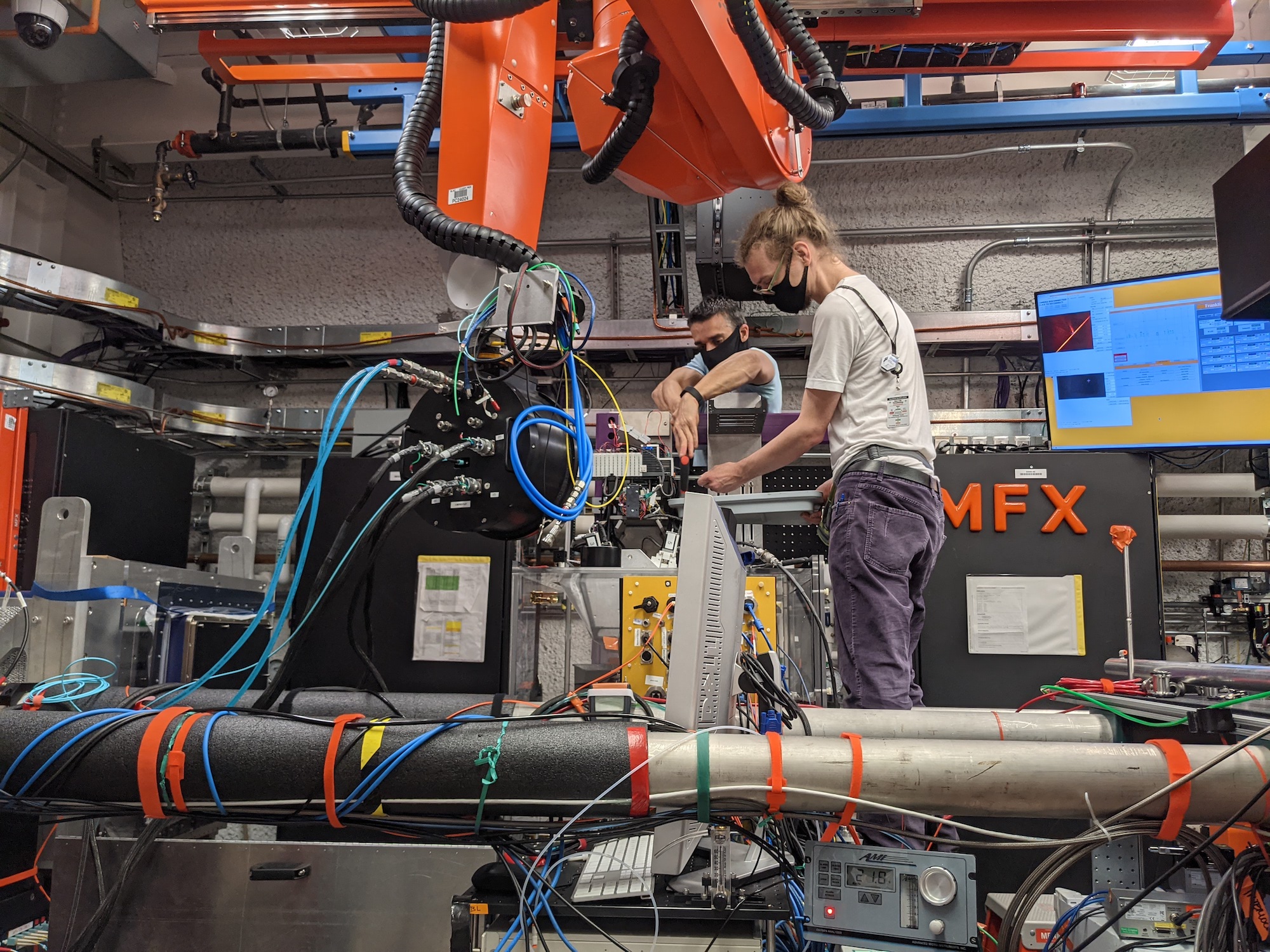
Philipp Simone, right, and Roberto Alonso-Mori, another author on the new study, set up equipment at the Macromolecular Femtosecond Crystallography instrument of the Linac Coherent Light Source, SLAC National Accelerator Laboratory. (Credit: Hiroki Makita/Berkeley Lab)
Q. It sounds like XFELs have a lot of advantages. Do they come with challenges?
Simon: The structural data recorded at X-ray free electron lasers is very complex, and only in collaboration with so many specialists one can obtain the final structure as we present it here. Also, my colleague Rana Hussein [co-first author from Humboldt University] did a fabulous job in carefully checking all the water positions in the really large channels present in the protein. But even then, the single puzzle pieces were really confusing at first, and it took us many rounds of glancing at them from all sides until the final picture emerged.
Q. Were there any surprises during the research?
Simon: Scientifically, definitely the moment when we saw for the first time the opening of the proton gate. The best moments, however, are, when we finish a successful X-ray beamtime session – in pre-pandemic times we had more than 20 people around – and all the faces are smiling… and then Junko [co-lead author Junko Yano] surprises us with ice cream.
Yachandra: The active site is not easily accessible to water, so it was clear that the enzyme was probably not using water directly from the outside of the membrane where PS II is located. While there are many potential channels in PS II, this study showed that one specific channel is involved in ferrying water from the outside, by determining the motion of water in the channel during the reaction, in real time.
Q. Philipp, why were you drawn to photosynthesis research?
Simon: I am a trained physicist specializing in optics and solid-state physics. After finishing my studies, I was looking for more applied research, where the outcome of my research could be directly embedded in a broader context. When I first read about the opportunity to study the function of photosynthetic proteins, I knew that’s what I want to work on. It combines my love for nature, the techniques I learned during my studies, and the outcome provides understanding of our immediate environment while also inspiring biomimicking technologies to harvest solar energy.
Q. Vittal, what motivates you?
Yachandra: I have been involved with photosynthesis research for a very long time now, and our research is driven by our goal to understand how plants split water using light in such a facile manner, while it is so difficult to mimic this reaction in artificial systems. It has been a lot of fun, working with our group members and our collaborators, and especially, Junko Yano and Jan Kern, co-leads of this paper and my longtime colleagues in MBIB, and Paul Adams and Nick Sauter, also from our Division.
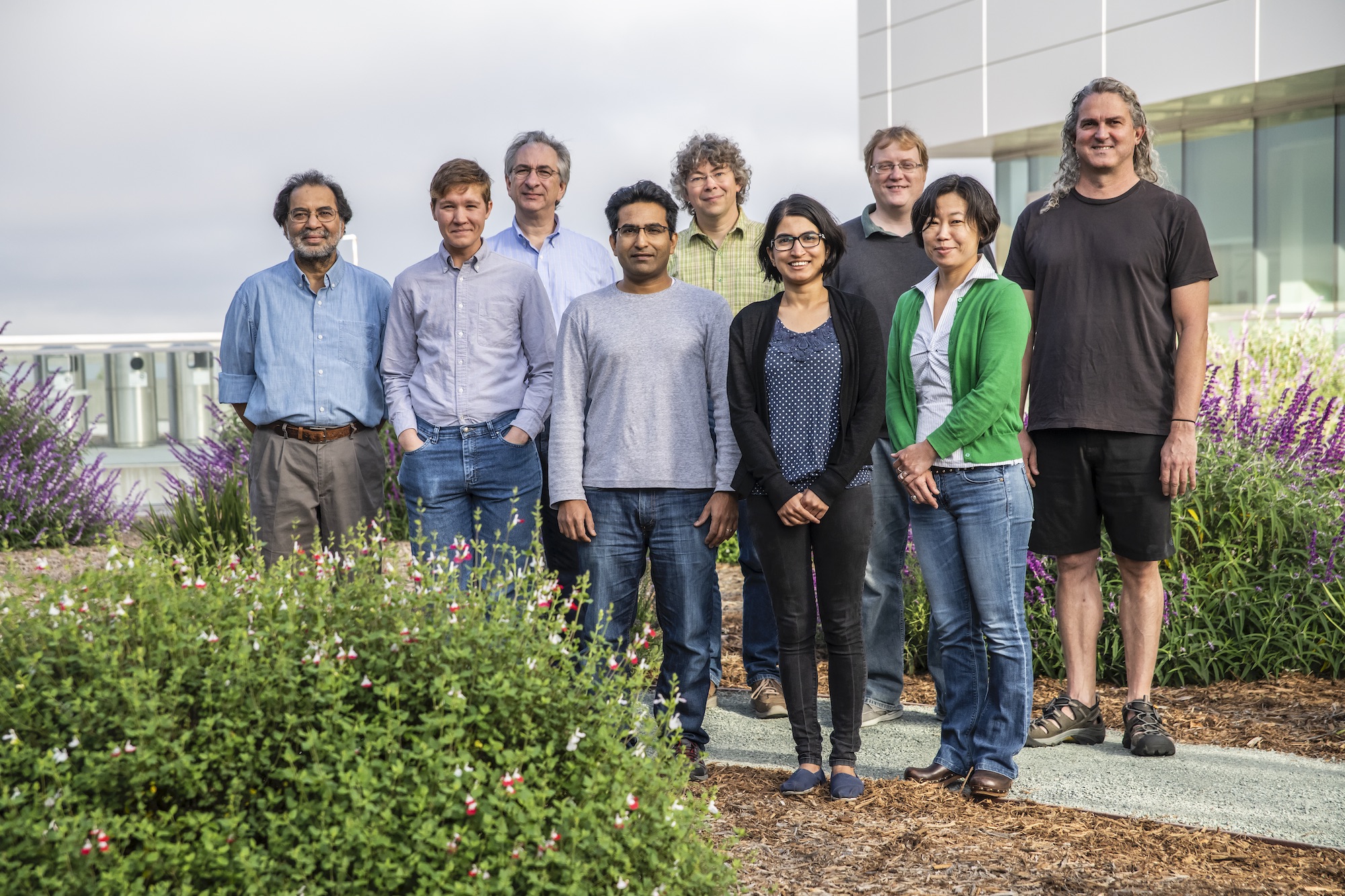
This photo, taken in 2018, shows many of the paper’s contributors. From left to right: Vittal Yachandra, Iris Young, Nicholas Sauter, Sheraz Gul, Jan Kern, Ruchira Chatterjee, Aaron Brewster, Junko Yano, and Nigel Moriarty, all in MBIB, within Berkeley Lab’s Biosciences Area. (Credit: Marilyn Sargent/Berkeley Lab)
This study included scientists from Berkeley Lab, Uppsala University, Humboldt University, SLAC, and University of Wisconsin–Madison.
# # #
Founded in 1931 on the belief that the biggest scientific challenges are best addressed by teams, Lawrence Berkeley National Laboratory and its scientists have been recognized with 14 Nobel Prizes. Today, Berkeley Lab researchers develop sustainable energy and environmental solutions, create useful new materials, advance the frontiers of computing, and probe the mysteries of life, matter, and the universe. Scientists from around the world rely on the Lab’s facilities for their own discovery science. Berkeley Lab is a multiprogram national laboratory, managed by the University of California for the U.S. Department of Energy’s Office of Science.
DOE’s Office of Science is the single largest supporter of basic research in the physical sciences in the United States, and is working to address some of the most pressing challenges of our time. For more information, please visit energy.gov/science.