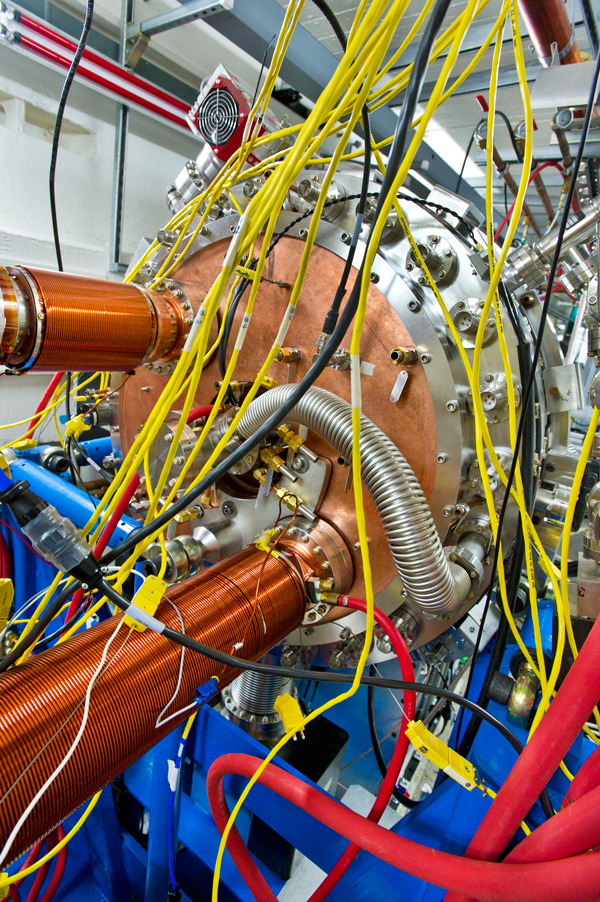
With its cables and wires and wave-guides and cables, APEX is a snug fit in the Beam Test Facility at the Advanced Light Source. (Lawrence Berkeley National Laboratory photo by Roy Kaltschmidt)
The focus of Berkeley Lab’s Advanced Photon Injector Experiment, APEX, is an extraordinary electron gun specially designed for the front end of superconducting accelerators. When it’s complete, the APEX gun will be able to produce well-formed bunches of electrons in pulses a few trillionths or even mere quadrillionths of a second long, at rates of up to a million bunches per second.
“With the kind of accelerator-based x-ray light sources we’re designing for, the quality of the electron beam is determined right when the electrons leave the gun,” says Fernando Sannibale of the Accelerator and Fusion Research Division (AFRD), who heads the APEX project. “We need tight electron bunches with high charge, high energy, and a very high repetition rate. And we need the gun to operate reliably over long periods.”
The APEX gun was conceived in 2006 by Sannibale and John Staples of AFRD and represents critical technology for the next generation of light sources. Initially funded under Berkeley Lab’s Laboratory Directed Research and Development program, since 2009 APEX has been supported by DOE’s Office of Science as part of a national program of research and development for accelerators and detectors.
A new kind of light source
In scientific parlance, a light source isn’t just a light bulb but a big machine that produces bright beams of light extending into the x-ray region. Typically light sources are user facilities, open to many scientists working on a wide range of experiments. Synchrotron light sources like Berkeley Lab’s Advanced Light Source (ALS), based on storage rings in which electrons circulate at high energies, are mainstays of scientific investigations in chemistry, complex materials, the life sciences, and other research frontiers.
The newest light sources are free electron lasers (FELs). Instead of synchrotrons, most FELs use linear accelerators to boost a train of electron bunches to relatively high energies, then send them through undulators, long rows of magnets that wiggle the electrons back and forth to make them shed much brighter x-ray beams than a synchrotron light source can achieve – and not just brighter, but coherent (laser-like) as well.
Unlike synchrotron sources, however, many FELs have tediously slow repetition rates. SLAC’s pioneering Linac Coherent Light Source (LCLS), for example, produces electron bunches, and thus x-ray pulses, about 100 times a second. Low rates don’t just glacially slow the time needed to do many experiments. Future experiments hope to explore the extreme time scales at which electrons move within molecules; these experiments will need very short x-ray pulses repeating hundreds of thousands or even a million times a second.
A video artist’s ultra-slow-motion impression of an APEX-style electron gun firing a continuous train of electron bunches into a superconducting linear accelerator (in reality this would happen a million times a second). As they approach the speed of light the bunches contract, maintaining beam quality. After acceleration, the electron bunches are diverted in one or more undulators, the key components of free electron lasers. Oscillating back and forth in the changing magnetic field, they create beams of structured x-ray pulses. Before entering the experimental areas the electron bunches are diverted to a beam dump. (Animation created by Illumina Visual for Lawrence Berkeley National Laboratory)
High-repetition-rate x-ray beams call for superconducting electron accelerators operating in what’s known as continuous-wave mode (CW). Rather than single pulses or groups of pulses separated by large time intervals, in CW a continuous electromagnetic field accelerates a train of short-spaced electron bunches to the FEL undulators without interruption. The oscillating voltage of the accelerating field is synchronized with the bunches in the train at constant radio frequency (RF).
For such an accelerator, the electron gun itself must operate in continuous wave mode at radio frequency, which poses a challenge. Producing a train of extremely short, fast-repeating, high-charge bunches, with the electrons in each bunch remaining tightly packed, requires a source of electrons that can spit them out rapidly and repeatedly in the presence of strong electric fields.
“The problem is that a high-frequency, high-power RF cavity can’t operate in CW; it has to be pulsed or it would melt,” says Sannibale. “So to operate in CW, we have to start with lower frequencies.”
The APEX gun operates at 187 megahertz (187 million cycles per second), or VHF – which means “very high frequency” but is low compared to the RF of the accelerator itself, measured in gigahertz (billions of cycles per second). The longer wavelength allows the gun’s cavity to be considerably larger than that of comparable higher-frequency guns, which means a greater surface area over which to distribute the heat, keeping the gun relatively cool while it accelerates a continuous wave of electron bunches.
The electron bunches come from a photocathode, a rod inside the gun that throws off electrons when hit by photons from a laser. The laser beam enters the gun’s beam-exit port in the opposite direction from the electron beam it stimulates, and is aligned closely parallel to it.
The first requirement for a photocathode that can produce a million bunches of electrons each second is high “quantum efficiency,” meaning it needs fewer photons to kick out each electron. The SLAC’s LCLS cathode, for example, is made of copper; it needs about 100,000 photons from a powerful laser to free a single electron. APEX is researching cathodes that will create high-charge bunches with just a hundred photons per electron.
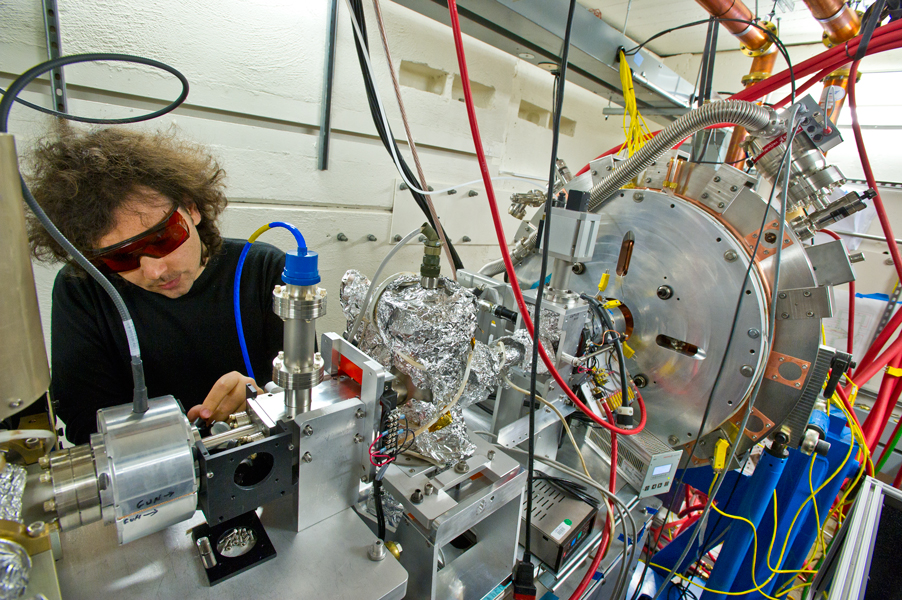
Daniele Filippetto, responsible for APEX’s photocathode laser and beam diagnostics, adjusts the mirror that directs the laser to fire into the gun, parallel to the emerging electron beam, to stimulate electron emission from the photocathode. (Lawrence Berkeley National Laboratory photo by Roy Kaltschmidt)
“The materials we’re most interested in are called multi-alkali antimonides, which can produce high-charge electron bunches at high rates; these photocathodes are being developed at Berkeley Lab by Howard Padmore’s group at the ALS,” says Sannibale. “The only catch is, the compounds are highly reactive. The slightest trace of water, oxygen, or carbon monoxide poisons them – so we have to get that kind of stuff out of the neighborhood.”
To suck everything out of the gun’s copper cavity it is ringed by slots, outside of which are mounted 24 pumps called “NEGs,” plus one vacuum ion pump. NEG stands for “nonevaporable getter,” a material that can react with and capture virtually everything except noble gases and some hydrocarbons, for which the ion pump is needed. The pumps is designed to pull the vacuum in the cavity down to a hard 10-10 Torr, as devoid of molecules as some regions of outer space.
Making electron bunches that are big enough and fast enough is only part of the answer to the challenge of creating a high-quality beam of continuous electron pulses that repeat a million times a second. Since the electrons all have the same charge, they try mightily to push one another apart, an effect called space charge. The trick is to minimize the electrical fields each can feel – the Coulomb forces they experience – without spreading them apart. Here’s where Einstein’s Special Theory of Relativity comes to the rescue.
“At a significant fraction of the speed of light, the electrons’ spherical fields start to flatten out – instead of a bunch of flying marbles they become a stack of flying pancakes, which reduces Coulomb forces in the longitudinal direction,” says APEX leader Fernando Sannibale. “The transverse direction, across the bunch, is a little more complex, but consider that a moving electric charge generates a magnetic field. Fast-moving electrons generate strong, attractive magnetic forces that hold the bunches together.”
Thus if the bunches can be accelerated to high energy and very high velocity at the moment they’re formed, they can maintain most of their compactness.
Other kinds of photocathodes will be tested as well, including cesium-telluride cathodes like those already in use at FLASH in Germany and other FELs; these are provided by Italy’s National Institute of Nuclear Physics (INFN) in Milan. Cesium-telluride cathodes are more robust and have quantum efficiency comparable to the multi-alkali antimonide cathodes, but require higher-energy photons to generate electron emission, and thus more powerful lasers.
Because the APEX gun is intended for advanced FEL user facilities, it has been fitted with a mechanism for unloading and reloading photocathodes relatively quickly (within an hour or two) without losing any of the gun’s vacuum.
Advancing a technological frontier
The APEX project is proceeding in three overlapping phases, starting with building the gun from scratch. In phase 0, now almost complete, the gun was designed by Russell Wells of the Engineering Division, fabricated in Berkeley Lab workshops, and installed in the Beam Test Facility at the Advanced Light Source. By December 15 of 2011 the RF fields were reliably performing at the nominal power, marking success for the project’s first fundamental milestone. Next the cathode load-lock mechanism will be installed; subsequently the gun’s vacuum performance will be verified.
Testing photocathode performance is already underway during the last stages of phase 0. As a first step, done in collaboration with a group at Brookhaven National Lab, the photocathode laser will be used to test a cathode amplifier made of diamond – not a source of electrons itself, but a way to multiply the number of electrons produced by a copper cathode three millimeters behind it; the performance of this promising diamond amplifier has never been measured in a RF gun, so the test will have scientific value in its own right. It’s also a good way to check the APEX system before tackling the challenges of multi-alkali antimonide cathodes.
Even as phase 0 is coming to an end, phase I has already begun. The emphasis is on rigorous proof of the photocathode candidates, the promising multi-alkali antimonides and the more robust cesium telluride. The other focus of phase I is testing beam quality at low energy, part of beam dynamics studies under postdoc Chris Papadopoulos.
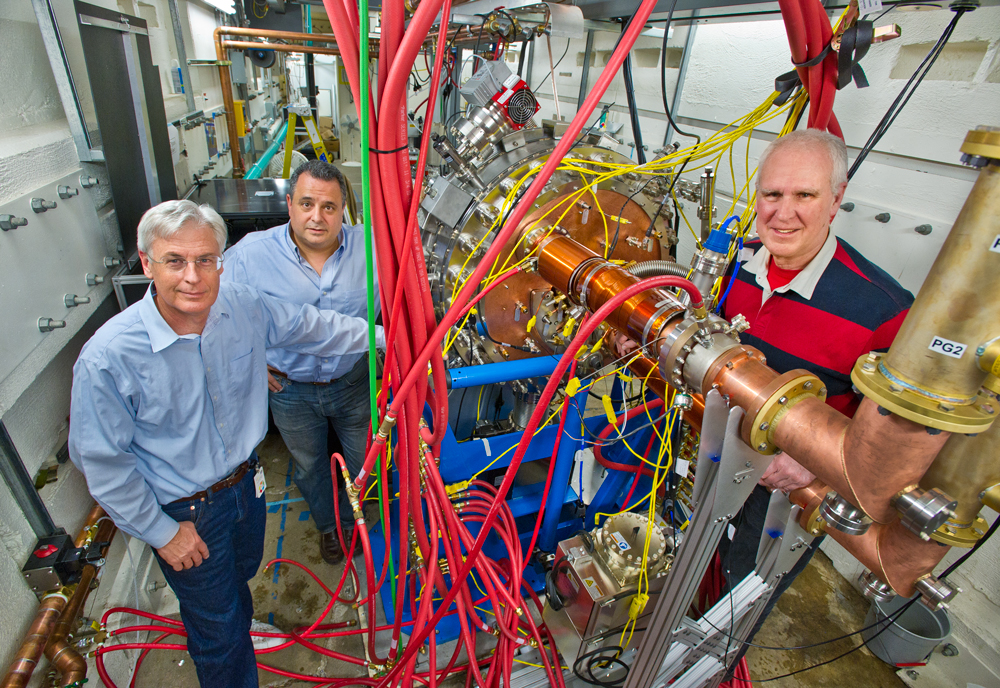
From right, John Staples and Fernando Sannibale of the Accelerator and Fusion Research Division conceived APEX, and Russell Wells (left) of Engineering led its construction. (Lawrence Berkeley National Laboratory photo by Roy Kaltschmidt)
After accelerating very quickly over a very short distance inside the gun, well-formed electron bunches (having low emittance, in accelerator-speak) leave the gun and enter the injector, the first stage of the accelerator itself. Since APEX is being tested in tight quarters – only three by 15 meters, with limited shielding – the bunches can be accelerated to at most 30 MeV (30 million electron volts), instead of the 70-100 MeV that will be required of a working injector operating in a FEL. During phase I the test won’t achieve even this energy: initially the beam will be kept at the energy of the bunches as they leave the gun, three quarters of an MeV.
However, says Sannibale, “We can only claim victory if we can demonstrate beam quality at higher energy.” That’s the main goal of phase II, which will test beam quality – emittance, bunch length, bunch charge, energy spread, and brightness – at 30 MeV. “Although we won’t be testing at the full energy required by a real injector, it will be high enough to show beam quality. Space charge forces will be sufficiently small to allow for a reliable characterization of the beam.”
Since its inception six years ago APEX has made significant progress. An electron gun that operates at radio frequency in continuous wave mode to produce a high-quality beam at an unprecedented repetition rate – it’s a concept that’s well on the road to final proof.
“APEX-style injectors will provide the front ends for a new generation of light sources, including Berkeley Lab’s own proposal for a facility with up to 10 FELs, 20 x-ray beamlines, and repetition rates up to a hundred thousand times a second for each. Each FEL will be independently tunable for wavelength, pulse length, polarization, and other experimental requirements,” Sannibale says. “APEX is the front end of an accelerator, but it’s at the forefront of a whole new way of doing photon science.”
###
APEX team members are Barry Bailey, Kenneth Baptiste, John Byrd, John Corlett, Carl Cork, Scott Dimaggio, Stefano De Santis, Lawrence Doolittle, Jennifer Ariel Doyle, Jun Feng, Daniele Filippetto, David Garcia Quintas, Gang Huang, Hanjing Huang, Rick Kraft, Toby Kramasz, Slawomir Kwiatkoswski, Richard Eric Lellinger, Michael Messerly, William Eric Norum, Howard Padmore, Christos Papadopoulos, Claudio Pellegrini, Conor Michael Pogue, Gregory Portmann, Matthew Prantil, Ji Qiang, Fernando Sannibale, John Staples, Mary Stuart, Theodore Vecchione, Massimiliano Vinco, Weishi Wan, Russell Wells, Max Zolotorev, and Frank Zucca.
The APEX project is a project principally of Berkeley Lab’s Advanced Light Source, Accelerator and Fusion Research, and Engineering Divisions and has benefited from the Lab’s Laboratory Directed Research and Development program, as well as from collaborations with Argonne National Laboratory, Brookhaven National Laboratory, Cornell University, Lawrence Livermore National Laboratory, the SLAC National Accelerator Laboratory, and the University of California at Berkeley.