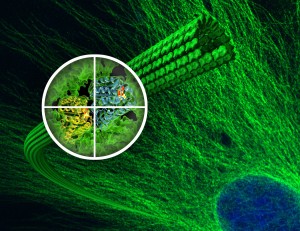
The most detailed look ever at the assembly and disassembly of microtubules, tiny fibers of tubulin protein that play a crucial role in cell division, provides new insight into the success of the anti-cancer drug Taxol.
A pathway to the design of even more effective versions of the powerful anti-cancer drug Taxol has been opened with the most detailed look ever at the assembly and disassembly of microtubules, tiny fibers of tubulin protein that form the cytoskeletons of living cells and play a crucial role in mitosis. Through a combination of high-resolution cryo-electron microscopy (cryo-EM) and new methodology for image analysis and structure interpretation, researchers with the Lawrence Berkeley National Laboratory (Berkeley Lab) and the University of California (UC) Berkeley have produced images of microtubule assembly and disassembly at the unprecedented resolution of 5 angstroms (Å). Among other insights, these observations provide the first explanation of Taxol’s success as a cancer chemotherapy agent.
“This is the first experimental demonstration of the link between nucleotide state and tubulin conformation within the microtubules and, by extension, the relationship between tubulin conformation and the transition from assembled to disassembled microtubule structure,” says Eva Nogales, a biophysicist with Berkeley Lab’s Life Sciences Division who led this research. “We now have a clear understanding of how hydrolysis of guanosine triphosphate (GTP) leads to microtubule destabilization and how Taxol works to inhibit this activity.”
Nogales, who is also a professor of biophysics and structural biology at UC Berkeley, as well as an investigator with the Howard Hughes Medical Institute, is the corresponding author of a paper describing this research in the journal Cell. The paper is entitled “High resolution αβ microtubule structures reveal the structural transitions in tubulin upon GTP hydrolysis.” Co-authors are Gregory Alushin, Gabriel Lander, Elizabeth Kellogg, Rui Zhang and David Baker.
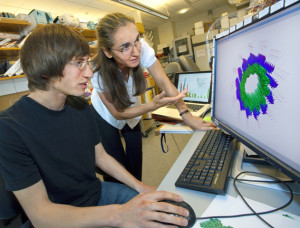
Gregory Alushin and Eva Nogales led a team of researchers that produced images of microtubule assembly and disassembly at the unprecedented resolution of 5 angstroms. (Photo by Roy Kaltschmidt)
During mitosis, the process by which a dividing cell duplicates its chromosomes and distributes them between two daughter cells, microtubules disassemble and reform into spindles across which the duplicate sets of chromosomes migrate. For chromosome migration to occur, the microtubules attached to them must disassemble, carrying the chromosomes in the process. The crucial ability of microtubules to transition from a rigid polymerized or “assembled” state to a flexible depolymerized or “disassembled” state – called “dynamic instability” – is driven by GTP hydrolysis in the microtubule lattice. Taxol prevents or dramatically slows down the unchecked cell division that is cancer by binding to a microtubule in such a manner as to block the effects of hydrolysis. However, until now the atomic details as to how microtubules transition from polymerized to depolymerized structures and the role that Taxol can play have been sketchy.
“Uncovering the atomic details of the conformational cycle accompanying polymerization, nucleotide hydrolysis, and depolymerization is essential for a complete description of microtubule dynamics,” Nogales says. “Such details should significantly aid in improving the potency and selectivity of existing anti-cancer drugs, as well as facilitate the development of novel agents.”
To find these details, Nogales, an expert in electron microscopy and image analysis and a leading authority on the structure and dynamics of microtubules, employed cryo-EM, in which protein samples are flash-frozen at liquid nitrogen temperatures to preserve their natural structure. Using an FEI 300 kV Titan cryo-EM from the laboratory of Robert Glaeser, she and her colleagues generated cryo-EM reconstructions of tubulin proteins whose structures were either stabilized by GMPCPP, a GTP analogue, or were unstable and bound to guanosine diphosphate (GDP), or were bound to GDP but stabilized by the presence of Taxol.
“To be able to distinguish the α-tubulin from the β-tubulin, we had to resolve our images at better than 8 Å, which most prior cryo-EM studies were unable to do,” Nogales says. “For that, we marked the subunits with kinesin, a protein motor that distinguishes between α- and β-tubulin.”
Nogales and her colleagues found that GTP hydrolysis and the release of the phosphate (GTP becomes GDP) leads to a compaction of the E-site and a rearrangement of the α-tubulin monomer that generates a strain on the microtubule that destabilizes its structure. Taxol binding leads to a reversal of this E-site compaction and α-tubulin rearrangement that restores structural stabilization.
“Remarkably, Taxol binding globally reverses the majority of the conformational changes we observe when comparing the GMPCPP and GDP states,” Nogales says. “We propose that GTP hydrolysis leads to conformational strain in the microtubule that would be released by bending during depolymerization. This model is consistent with the changes we observe upon taxol binding, which dramatically stabilizes the microtubule lattice. Our analysis supports a model in which microtubule-stabilizing agents like Taxol modulate conformational strain and longitudinal contacts in the microtubule lattice.”
This research was supported by NIH’s National Institute of General Medical Sciences, the Damon Runyon Cancer Research Foundation, and the Howard Hughes Medical Institute.
###
Lawrence Berkeley National Laboratory addresses the world’s most urgent scientific challenges by advancing sustainable energy, protecting human health, creating new materials, and revealing the origin and fate of the universe. Founded in 1931, Berkeley Lab’s scientific expertise has been recognized with 13 Nobel prizes. The University of California manages Berkeley Lab for the U.S. Department of Energy’s Office of Science. For more, visit www.lbl.gov.
Additional Information
For more about the research of Eva Nogales go here
A UC Berkeley release on this work features two short videos that illustrate Taxol assembly and disassembly. The release can be viewed here